
I. Grinding and Grinding Machines
1. Grinding
Grinding is a commonly used semi-finishing and finishing method. Compared to other machine tools, the main difference lies in the cutting tool used. Grinding uses high-speed rotating grinding wheels, vibrating grinding stones, or rotating abrasive belts to process the workpiece surface. Grinding is mainly used for processing various internal and external cylindrical surfaces, internal and external conical surfaces, flat surfaces, as well as special and complex formed surfaces such as threads, gears, and splines.
A grinding machine is a machine tool that uses abrasives to grind the surface of workpieces. Most grinding machines use high-speed rotating grinding wheels for grinding, while a few use other abrasives such as grinding stones, abrasive belts, and loose abrasives for processing, such as honing machines, superfinishing machines, belt grinders, lapping machines, and polishing machines.
The grinding wheel is the main cutting tool for grinding and serves as the cutting tool of the grinding machine. Due to the high hardness of the abrasive grains, the grinding wheel has self-sharpening properties. Grinding can be used to process various materials, including hardened steel, high-strength alloy steel, carbides, non-ferrous metals (non-iron materials), glass, ceramics, and marble, as well as other high-hardness metallic and non-metallic materials.
Grinding speed refers to the linear speed of the grinding wheel. For ordinary grinding, the linear speed of the grinding wheel is generally 30-35 m/s. For high-speed grinding, the linear speed is typically 45-160 m/s. When it exceeds 160 m/s, it is called ultra-high-speed grinding.
Grinding is usually used for semi-finishing and finishing. The dimensional tolerance grade can reach IT8 to IT5 or even higher. The surface roughness for general grinding is Ra 1.25-0.16 μm, for precision grinding is Ra 0.16-0.04 μm, for ultra-precision grinding is Ra 0.04-0.01 μm, and mirror grinding can achieve below Ra 0.01 μm.
The specific energy consumption (or specific energy, which is the energy consumed to remove a unit volume of workpiece material) for grinding is higher than that of general cutting, and the metal removal rate is lower than general cutting. Therefore, before grinding, workpieces usually undergo other cutting methods to remove most of the machining allowance, leaving only 0.1-1 mm or less grinding allowance.
With the development of high-efficiency grinding techniques such as creep feed grinding and high-speed grinding, it is now possible to grind parts directly from raw materials. Grinding is also used for rough machining, such as removing casting gates and risers, forging flash, and steel ingot skins.
2. Development of grinding machines
In the 1730s, to meet the needs of processing hardened parts for clocks, bicycles, sewing machines, and firearms, grinding machines using natural abrasive wheels were developed in England, Germany, and the United States.
These grinding machines were modified from existing machine tools such as lathes and planers by adding grinding heads. They had simple structures, low rigidity, and were prone to vibration during grinding. Operators needed to have very high skills to grind precise workpieces.
In 1876, the universal cylindrical grinding machine manufactured by the American Brown & Sharpe Company, exhibited at the Paris Exhibition, was the first to have the basic features of modern grinding machines. Its workpiece headstock and tailstock were mounted on a reciprocating worktable, the box-shaped bed increased machine rigidity, and it came with an internal grinding attachment. In 1883, this company produced a surface grinding machine with the grinding head mounted on a column and a reciprocating worktable.
Around 1900, the development of artificial abrasives and the application of hydraulic transmission greatly promoted the development of grinding machines. With the development of modern industry, especially the automotive industry, various types of grinding machines emerged. For example, in the early 20th century, planetary internal grinding machines for cylinder blocks, crankshaft grinding machines, camshaft grinding machines, and piston ring grinding machines with electromagnetic chucks were successively developed.
Automatic measuring devices began to be applied to grinding machines in 1908. By the 1920s, centerless grinding machines, double-face grinding machines, roll grinding machines, guideway grinding machines, honing machines, and superfinishing machines were successively developed and put into use.
In the 1950s, high-precision external cylindrical grinding machines capable of mirror grinding appeared; in the late 1960s, high-speed grinding machines with grinding wheel linear speeds of 60-80 m/s and surface grinding machines with large depth of cut and slow feed emerged; in the 1970s, technologies such as digital control and adaptive control using microprocessors were widely applied in grinding machines.
With the increase in the number of high-precision and high-hardness mechanical parts, as well as the development of precision casting and precision forging technologies, the performance, variety, and production of grinding machines have been continuously improving and increasing.
Grinding machines are the most diverse category among all types of metal cutting machine tools. The main types include external cylindrical grinding machines, internal grinding machines, surface grinding machines, centerless grinding machines, and tool grinding machines.
- External cylindrical grinding machines are the basic series of general types, mainly used for grinding cylindrical and conical external surfaces.
- Internal grinding machines are the basic series of general types, mainly used for grinding cylindrical and conical internal surfaces. In addition, there are grinding machines that can perform both internal and external grinding.
- Jig grinding machines are internal grinding machines with precision coordinate positioning devices.
- Centerless grinding machines hold workpieces without centers, generally supported between a regulating wheel and a work rest blade. The regulating wheel drives the workpiece to rotate, mainly used for grinding cylindrical surfaces.
- Surface grinding machines are mainly used for grinding flat surfaces of workpieces.
- Belt grinding machines are grinding machines that use fast-moving abrasive belts for grinding.
- Honing machines are grinding machines used for honing various surfaces of workpieces.
- Lapping machines are grinding machines used for lapping flat surfaces or cylindrical internal and external surfaces of workpieces.
- Guideway grinding machines are mainly used for grinding machine tool guideways.
- Tool grinding machines are used for grinding tools.
- Multi-purpose grinding machines are used for grinding cylindrical, conical internal and external surfaces or flat surfaces, and can grind various workpieces using following devices and attachments.
- Special-purpose grinding machines are dedicated machine tools for grinding specific types of parts. They can be further classified according to their processing objects: spline shaft grinding machines, crankshaft grinding machines, cam grinding machines, gear grinding machines, thread grinding machines, curve grinding machines, etc.
3. Basic characteristics of grinding
(1) Grinding can achieve high processing accuracy and low surface roughness values.
Dimensional tolerance grades can reach IT6 and above; surface roughness values range from Ra 0.8 to 0.01 μm, and for mirror grinding, it can be Ra 0.01 μm and above. Grinding can not only be used for finishing but also for rough grinding, coarse grinding, and heavy-load grinding.
(2) The radial grinding force in grinding is large.
The radial grinding force (back force) in grinding is large and acts in the direction where the technological system has lower rigidity, which can cause elastic deformation of the workpiece, fixture, and machine tool, affecting processing accuracy.
Therefore, when processing workpieces with lower rigidity (such as grinding slender shafts), appropriate measures should be taken to prevent workpiece deformation from affecting processing accuracy.
(3) The high cutting speed in grinding leads to high grinding temperatures.
From experimental measurements, the grinding temperature (average working temperature) at a grinding wheel linear speed of 60 m/s is 50%-70% higher than at 30 m/s; at 80 m/s, the grinding temperature is 15%-20% higher than at 60 m/s. This is because as the grinding wheel linear speed increases, the number of abrasive grains participating in grinding per unit time increases, friction intensifies, and energy consumption also increases, raising the temperature of the workpiece surface layer.
Research shows that 80%-90% of the heat generated during grinding is transferred to the workpiece (10%-15% to the grinding wheel, 1%-10% carried away by grinding chips). Coupled with the poor thermal conductivity of the grinding wheel, this can easily cause workpiece surface burning and microcracks.
Therefore, large amounts of cutting fluid with high cooling efficiency should be used during grinding to reduce grinding temperature. In high-speed grinding, the cutting fluid is often separately cooled to achieve better cooling effects during operation.
(4) Grinding wheels have a self-sharpening effect.
During the grinding process, the fracturing of abrasive grains produces new, sharper edges, and the shedding of abrasive grains exposes a new layer of sharp grains, allowing the grinding wheel to partially recover its cutting ability. This phenomenon is called the self-sharpening effect of the grinding wheel, which is beneficial for grinding and is not found in other cutting tools.
When grinding, the self-sharpening effect of the grinding wheel is often utilized to improve grinding efficiency by appropriately selecting the hardness of the grinding wheel. However, the uneven shedding of abrasive grains can cause the grinding wheel to lose its shape accuracy; broken abrasive grains and chips can clog the grinding wheel. Therefore, after grinding for a certain period, the grinding wheel needs to be dressed to restore its cutting ability and shape accuracy.
(5) Grinding has a wide range of technological applications.
In addition to processing general structural materials such as cast iron, carbon steel, and alloy steel, grinding can also process high-hardness materials that are difficult to cut with general tools, such as hardened steel, carbides, ceramics, and glass. However, it is not suitable for finish machining non-ferrous metal workpieces with high plasticity. Grinding can not only process various surfaces such as external cylindrical surfaces,
internal cylindrical surfaces, flat surfaces, formed surfaces, threads, and gear profiles, but is also commonly used for sharpening various cutting tools.
II. Types of grinding processes and grinding characteristics
1. Classification of grinding processes
Grinding processes are usually classified according to the type of grinding tool, divided into two main categories: fixed abrasive processing and loose abrasive processing, as shown in Figure 1.
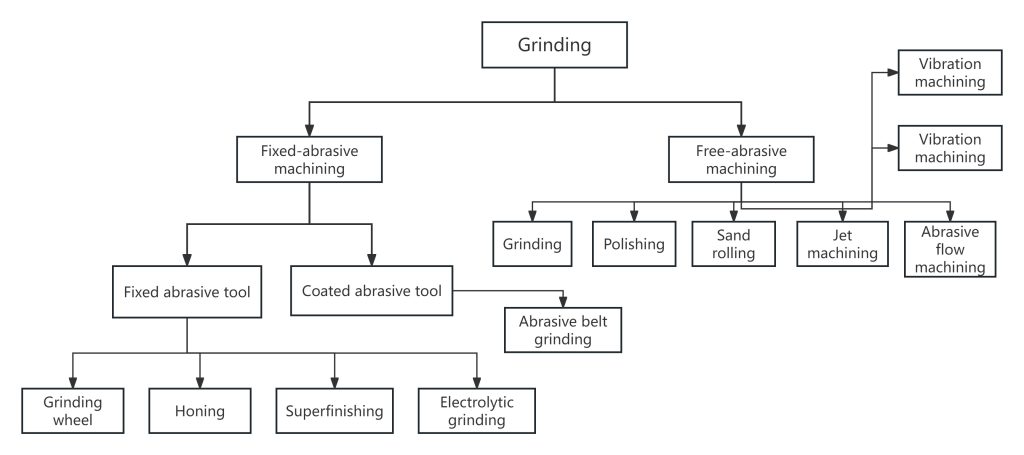
Different forms of grinding processes have significant differences in their applications, working principles, and movement patterns. They can generally be classified according to the processing object into external cylindrical, internal cylindrical, surface, and form grinding, as shown in Figure 2 and Table 1. However, all grinding processes involve friction, micro-cutting, and surface chemical-physical reactions, differing only in form and degree. The term “grinding” usually refers mainly to grinding using a grinding wheel.
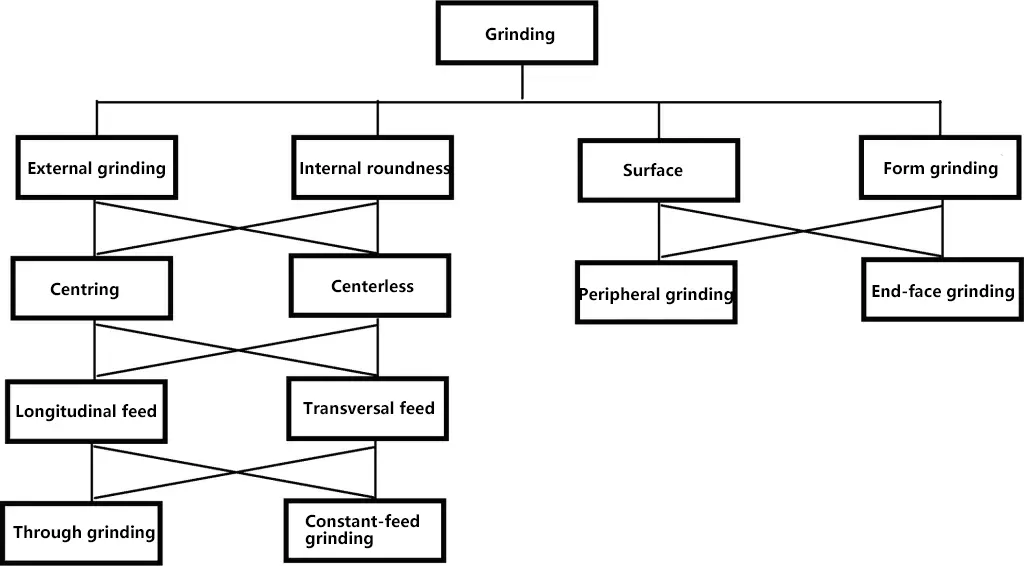
Table 1 Basic Grinding Methods
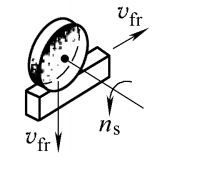
General rotational surfaces (internal and external) can be classified into center grinding and centerless grinding based on the method of clamping and driving the workpiece; based on the relationship between the feed direction and the machined surface, they can be divided into longitudinal feed grinding and transverse feed grinding; considering the position of the grinding wheel relative to the workpiece after the grinding stroke, they can be further divided into through grinding and plunge grinding; based on the type of grinding wheel working surface, they can be classified into peripheral grinding, face grinding, and peripheral-face grinding.
The grinding methods and techniques shown in Figure 2 should be selected based on specific conditions. For example, when grinding a plane surface, either face grinding or peripheral grinding can be used, depending on the equipment, processing conditions, and processing habits.
There are two basic purposes for grinding with abrasive wheels: one is to machine workpieces to certain dimensions and shapes for semi-finishing and finishing, which is a machining method for hardened steel and various high-hardness, high-strength, difficult-to-machine alloy materials, as well as for non-metallic materials such as ceramics, optical glass, rubber, and wood.
The other purpose is to quickly remove large amounts of material in rough grinding at the lowest cost, such as heavy-duty grinding on steel billets to remove surface defect layers, where the material removal rate can reach hundreds of kilograms per hour, accounting for 3% to 7% of the total billet mass.
In recent years, abrasive belt grinding has been gradually promoted, showing a trend of replacing grinding wheels in some aspects. Its advantages include high grinding efficiency, simple equipment, low cost, and strong adaptability, earning it the title of “universal grinding”.
From the above, it can be seen that grinding, in a broad sense, refers to machining using fixed abrasive tools. However, in this book, grinding specifically refers to machining using high-speed rotating grinding wheels, which is grinding in the narrow sense.
2. Characteristics of Grinding
Grinding is a general term for machining using abrasive grains and abrasive tools. Compared to cutting processes such as turning and milling, it has the following characteristics:
(1) The cutting edges on the grinding wheel surface are very hard mineral abrasive grains
Their shape, size, and distribution are in a random state. Due to differences in abrasive tool properties and grinding conditions, the actual grinding grains on the wheel surface account for 10% to 50% of the total number of grains on the surface. Currently, diamond abrasive grains and cubic boron nitride (CBN) grains are also being applied.
(2) The cutting thickness of each abrasive grain in grinding is very thin
Generally only 1-10μm, therefore high accuracy and low surface roughness can be achieved on the machined surface. Typically, dimensional tolerance grades of IT6-IT7 can be achieved, with surface roughness values of Ra0.1-0.05μm. For mirror grinding, surface roughness values can reach Ra0.04-0.01μm. Thus, the accuracy and surface roughness obtained through grinding are superior to other cutting processes.
(3) The cutting speed of abrasive grains is high.
In general grinding, the linear speed of the grinding wheel is 35-60m/s, which is more than 20 times that of ordinary cutting tools, allowing for higher metal removal rates. Currently, using different abrasive tools, grinding wheel linear speeds can reach 120-300m/s for ultra-high-speed grinding, making it possible to process materials with high elasticity. Even though each individual chip is very small, the overall processing efficiency can be improved.
(4) Grinding wheel abrasive grains have high hardness and good thermal stability.
They can not only grind steel, cast iron, and other materials, but also grind various high-hardness materials such as hardened steel, carbides, glass, ceramics, and stone. These materials are difficult to machine using conventional turning, milling, and other methods.
(5) Abrasive grains have a certain brittleness.
During grinding, worn cutting edges at the front of the abrasive grains will fracture and break under excessive grinding forces, creating new sharp cutting edges. This is known as the “self-sharpening effect” of grinding wheels.
(6) It is easy to dress and sharpen grinding wheels.
The abrasive cutting edges of grinding wheels have very high hardness but also possess a certain brittleness, making it easy to dress and sharpen the abrasive cutting edges. Even for diamond grinding wheels, due to their low grain brittleness, using softer metal binders like copper alloys or resin binders makes it possible to dress the diamond wheels while machining highly brittle materials like glass, as the binder is destroyed and the grains fall off.
(7) The temperature at the grinding point is high.
A certain amount of energy is required to remove a unit volume of chips, and the smaller the chip size, the more energy is needed. Strong friction and rapid plastic deformation occur between the abrasive grains and the workpiece, generating a large amount of grinding heat, with grinding temperatures reaching 1000-1500°C. Therefore, a large amount of cutting fluid is needed for cooling during grinding.
3. Basic Grinding Parameters
During the grinding process, the grinding wheel and workpiece move relative to each other, as briefly classified in Figure 3.
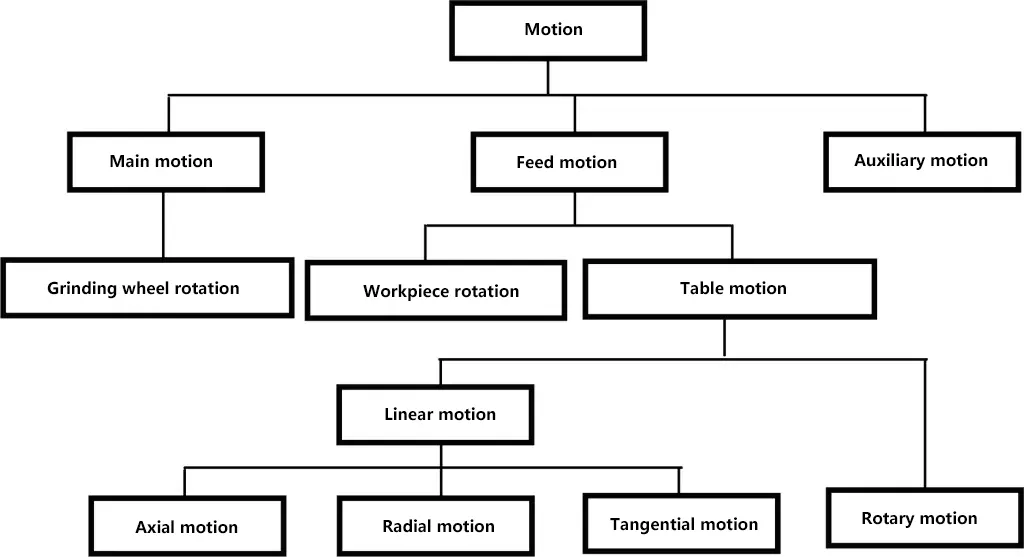
There are many grinding methods, commonly including external cylindrical grinding, internal cylindrical grinding, surface grinding, form grinding, thread grinding, and gear grinding. However, based on the working surface of the grinding wheel, they can be basically classified into peripheral grinding, face grinding, and form grinding, as shown in Figure 2 and Table 1. According to the various movements of different grinding methods, they can be summarized into two types: primary motion and feed motion.
(1) Primary Motion
The motion that directly removes metal from the workpiece and turns it into chips is called the primary motion. In grinding, the rotational motion of the grinding wheel is the primary motion. The primary motion speed is high and consumes most of the machine tool’s power.
The tangential speed at the maximum diameter of the grinding wheel is the grinding speed vs. That is
vs=πdsns/1000×60
Where
- vs – grinding speed (m/s);
- ds – grinding wheel diameter (mm);
- ns – grinding wheel rotation speed (r/min).
The grinding speed for external cylindrical and surface grinding is generally 35-60m/s, while for internal grinding it is usually 15-30m/s. From the above equation, it can be seen that as the grinding wheel diameter decreases due to wear, the grinding speed will decrease, affecting grinding quality and production efficiency. Therefore, when the grinding wheel diameter reduces to a certain value, the wheel should be replaced or the wheel speed should be increased to ensure a reasonable grinding speed.
(2) Feed Motion
The motion that continuously feeds the metal to be cut into the cutting process to gradually cut out the entire workpiece surface is called the feed motion.
In external cylindrical grinding, the rotational motion of the workpiece around its own axis is the workpiece’s circular feed motion; the reciprocating linear motion of the workpiece is the workpiece’s axial feed motion (also called longitudinal feed motion); the transverse motion of the grinding wheel is the wheel’s radial feed motion (also called transverse feed motion).
1) Workpiece rotational motion
The calculation formula for the workpiece circumferential feed motion speed vw (m/min) is
vw=πdwnw/1000
Where
- dw – workpiece diameter (mm);
- nw – workpiece rotation speed (r/min).
The workpiece circumferential speed is generally 10-30m/s, chosen according to processing requirements. For higher precision, a lower speed can be selected; otherwise, a higher speed can be chosen. In actual production, the workpiece speed is often selected first, then the workpiece rotation speed is calculated to adjust the machine tool speed. For this purpose, the above formula can be transformed into
nw=1000vw/πdw≈318vw/dw
2) Axial feed motion
Axial feed motion refers to the movement of the worktable in the direction parallel to the grinding wheel axis. The axial feed amount (longitudinal feed amount) refers to the movement of the worktable relative to the grinding wheel axis for each workpiece rotation (or each stroke), denoted as fa (mm/r).
The axial feed amount is limited by the width of the grinding wheel and can be calculated using the following formula when selecting
fa=(0.1~0.8)B
Where B is the width of the grinding wheel (mm).
The calculation formula for the worktable axial feed speed (mm/min) is
vfa=nwfa
3) Radial feed motion
The radial feed amount of the grinding wheel slide in the direction perpendicular to the worktable refers to the displacement of the radial feed by the grinding wheel slide for each workpiece rotation (or each stroke), denoted as f. The radial feed motion is generally discontinuous, with the grinding wheel only feeding radially at the end of each workpiece stroke. Therefore, the radial feed amount f is expressed in mm/single stroke or mm/double stroke.
For external cylindrical grinding, the infeed amount, also known as the transverse feed amount, refers to the distance the grinding wheel moves transversely at the end of each longitudinal reciprocating stroke of the worktable. A larger infeed amount results in higher productivity but is unfavorable for improving grinding accuracy and reducing surface roughness values.
The infeed amount ap refers to the grinding wheel cutting depth measured perpendicular to the workpiece surface, so
ap=(D-d)/2
Where
- D – workpiece diameter before feeding (mm);
- d – workpiece diameter after feeding (mm).
Typically, for rough grinding of external cylinders, ap=0.01-0.025mm; for finish grinding of external cylinders, ap=0.005-0.015mm, with smaller values for finish grinding and larger values for rough grinding.
For modern high-speed external cylindrical grinding machines, rough grinding of external cylinders uses ap=0.05-0.5mm; finish grinding of external cylinders uses ap=0.005-0.015mm.
(3) Contact arc length lg between grinding wheel and workpiece
The calculation formula for lg is
lg≈√(apDs)
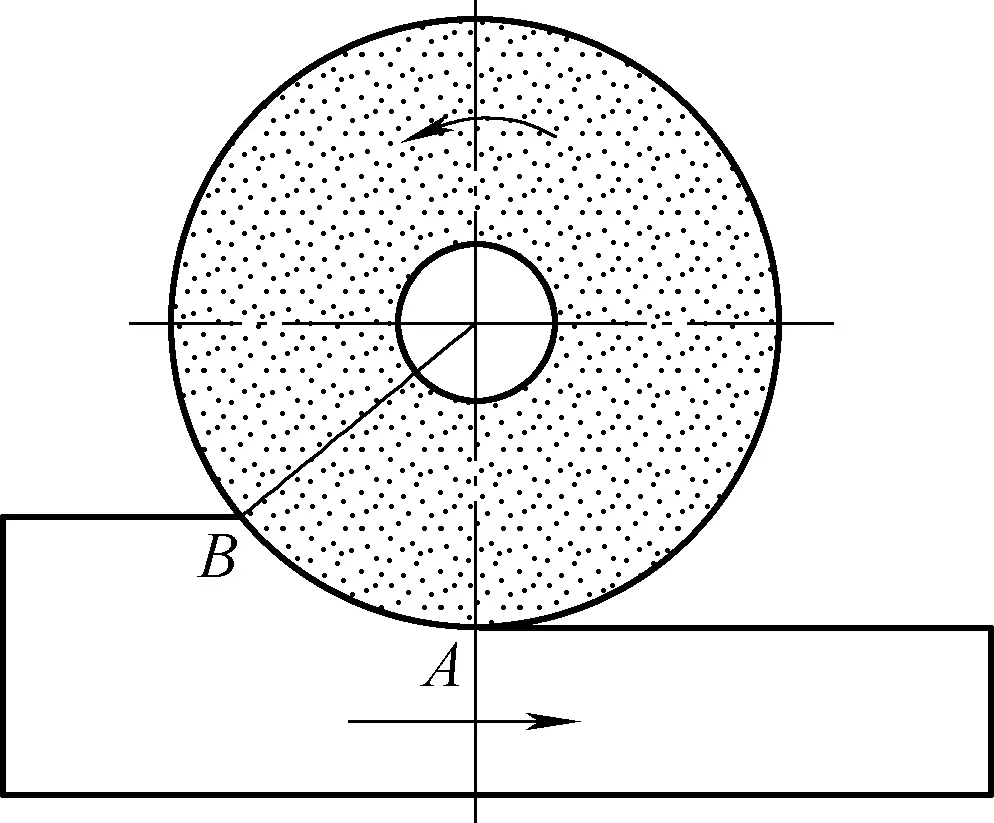
As shown in Figure 4, the size of lg indicates the size of the heat source. The larger lg is, the larger the grinding heat source, making cooling and chip removal difficult, and the grinding wheel is prone to clogging. Generally, internal cylindrical grinding has the longest contact arc length, followed by surface grinding, with external cylindrical grinding being the smallest.
(4) Average grinding thickness acg
The calculation formula for average grinding thickness acg is
acgmax=2vwlx/vs√[(1/dw±1/Ds)fr]
In the formula, the smaller the vw/vs value, the smaller acgmax will be, so high-speed grinding is beneficial; acgmax is proportional to √fr, when the grinding depth fr increases by 1 time, acgmax only increases by about 40%, from the perspective of increasing production efficiency, increasing fr (deep grinding method) is more beneficial than increasing vw; when the distance between abrasive grains lx decreases (fine grain size), acgmax also decreases, so finer abrasive grains are used for fine grinding; increasing Dw and Ds can reduce acgmax.
(5) Material removal rate Qw
The volume of workpiece material ground per unit time is called the material removal rate (mm3/min). Its calculation formula is
Qw=1000vwfrap
The material removal rate per unit effective grinding wheel width Q’w (mm3/mm·min) is
Q’w=Qw/b
Where b is the effective grinding width of the wheel (mm).
Q’w indicates the grinding performance and productivity of the wheel. A high Q’w value indicates good grinding performance and high production efficiency.
(6) Grinding ratio G
The calculation formula for grinding ratio G is
G=Qw/Qs
Where Qs represents the volume of grinding wheel material falling off per minute, also known as the grinding wheel wear rate. A large G value indicates good cutting performance of the wheel, high production efficiency, and good economic effect.
The calculation formula for wear ratio G is
Gs=1/G=Qs/Qw
A small Gs value indicates long wheel life and good economic effect.
(7) Specific grinding energy Uc
The energy consumed to remove a unit volume (or mass) of material, with units of N·m/mm3 or J/mm3. Uc is commonly used to estimate grinding force and power. A smaller Uc indicates better grindability of the material and relatively high metal removal rate.
III. Grinding forces and grinding heat
1. Grinding process
The essence of grinding processing is that the metal surface layer of the workpiece is ground under the instantaneous compression, scribing, cutting, and friction polishing of countless abrasive grains. The contact between abrasive grains and the workpiece during grinding is shown in Figure 5. The chip formation process during grinding can be roughly divided into the following three stages:
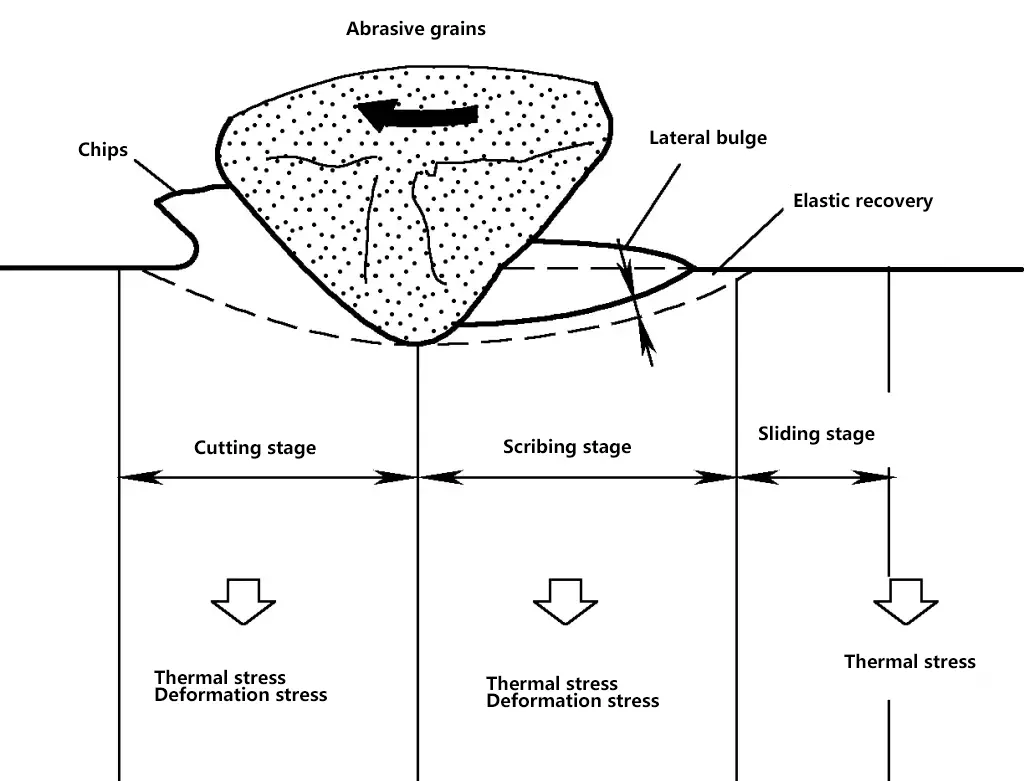
Stage I: The abrasive grain begins to contact the workpiece, but does not cut into it, only causing friction on the surface, producing thermal stress in the surface layer of the workpiece. This stage is called the sliding friction stage.
Stage II: As the cutting depth of the abrasive grain increases, it gradually cuts into the workpiece, causing the material to bulge on both sides, forming grooves on the workpiece surface. The workpiece enters the second stage of plastic deformation. At this time, in addition to friction between the abrasive grain and the workpiece, more importantly, internal friction of the material and stress caused by elastic deformation occur. This stage affects the surface roughness of the workpiece and surface defects such as burns and cracks. This stage is called the scribing stage.
Stage III: At this point, the abrasive grain has cut to a certain depth, and the normal cutting force has increased to a certain level. The cut area has also reached a certain temperature, causing the grains in this part of the material to slip, continuously increasing plastic deformation. When the grinding force reaches the strength limit of the workpiece, the ground layer material experiences crushing, and the material slides along the shear plane to form chips, producing thermal stress and deformation stress in the surface layer of the workpiece. This stage is called the cutting stage.
The grinding process is characterized by the effects of force and heat. During the grinding process, some protruding and sharp abrasive grains cut off a certain thickness of metal under the action of compression and friction; while duller grains only cause surface scribing; low-protruding and dulled grains, or the latter of two adjacent grains, only produce friction. The chips cut by the abrasive grains are very small (except for heavy-duty grinding), generally divided into ribbon-like chips, fragmented chips, and molten spherical chips.
2. Grinding forces and grinding power
(1) Grinding force
During grinding, friction and chip deformation occur between the grinding wheel and the workpiece, resulting in equal and opposite forces acting on the grinding wheel and workpiece, called grinding forces, as shown in Figure 6.
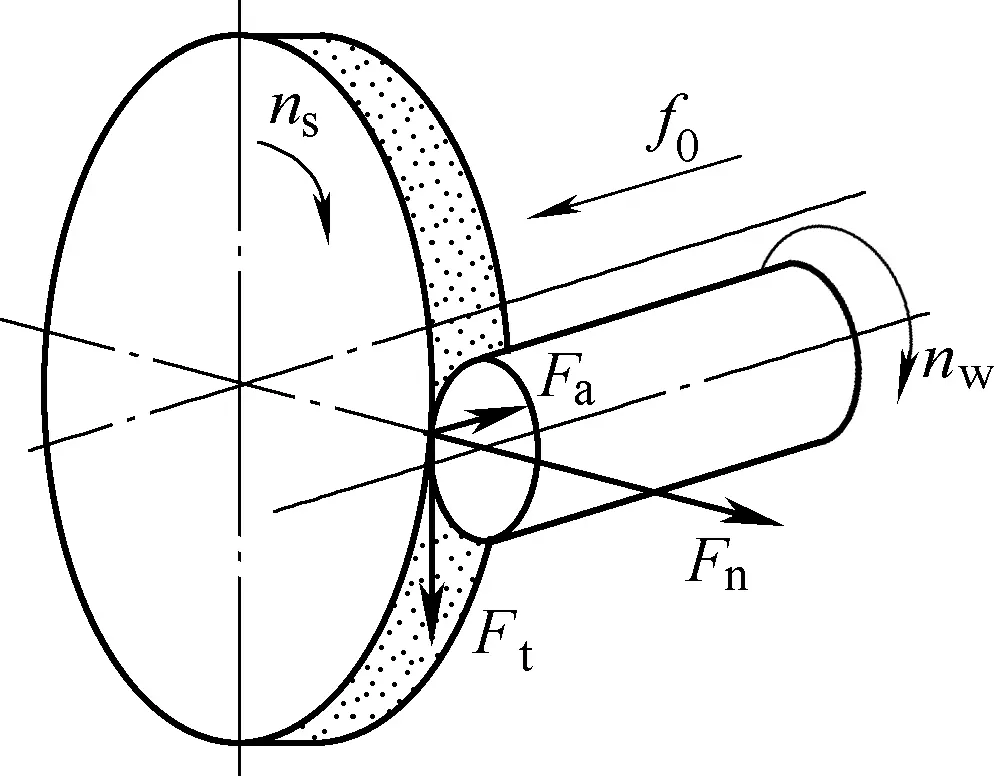
- Ft—Tangential grinding force
- Fn—Normal grinding force
- Fa—Axial grinding force
In general external cylindrical grinding, the grinding force can be decomposed into three mutually perpendicular components: tangential grinding force Ft (in the direction of the grinding wheel’s rotational circumference tangent); normal grinding force Fn (in the direction normal to the contact surface between the grinding wheel and workpiece, also called radial component); axial grinding force Fa (in the direction of longitudinal feed).
Generally, the ratio of the three grinding force components is: Fa=(0.1~0.2)Ft, Fn=(1.5~3.2)Ft. From this, we can see that Fn is the largest and Fa is the smallest. Ft is the main parameter for determining the power of the grinding machine’s motor and is also called the main grinding force. Fn mainly causes deformation of the grinding wheel and workpiece, accelerates wheel dulling, and directly affects the machining accuracy and surface quality of the workpiece. Fa acts on the feed system of the grinding machine, but its value is very small compared to Ft and can generally be ignored.
Grinding force can be measured with a dynamometer or calculated using empirical formulas (refer to relevant manuals). In production, it can also be calculated based on the actual input power of the motor F(N). That is
Ft=PEηE/πnsDs×106
Where
- PE – Measured input power of the grinding head motor (kW);
- ηE – Motor transmission efficiency;
- ns – Grinding wheel speed (r/min);
- Ds – Grinding wheel diameter (mm).
This method is suitable for various grinding modes but can only determine the average value of the tangential grinding force.
(2) Grinding power
The grinding power Pm is the basis for designing or verifying the power parameters of a grinding machine. Due to the high wheel speed and large power consumption, verification is necessary when required. The power consumed by the main motion, i.e., the grinding power Pm (kW), is
Pm=Ftvs/1000
The grinding wheel motor power Ph is calculated by the following formula
Ph=Pm/ηm
Where ηm is the total mechanical transmission efficiency of the grinding machine, generally ηm = 0.70 to 0.85.
3. Grinding heat and grinding temperature
During grinding, due to high cutting speed, small cutting thickness, and blunt abrasive grains, the power consumption during cutting is very high, about 10 to 20 times that of turning or milling. Most of the consumed energy is converted into heat. About 80% of this heat is transferred to the workpiece (compared to only 3% to 9% in turning), 4% to 8% is carried away by chips, 10% to 16% is taken away by the grinding wheel, and a small portion is dissipated through conduction and radiation.
Due to the high grinding speed, the heat doesn’t have time to penetrate deep into the workpiece and instantly accumulates on the surface layer. The temperature below the surface layer (about 1mm deep) is only a few dozen degrees, thus creating a large temperature gradient. When the surface layer temperature is very high, burns and thermal deformation may occur on the machined surface, affecting the surface quality and machining accuracy. Therefore, controlling and reducing the grinding temperature is a crucial step in ensuring grinding quality.
Due to the large temperature differences near the grinding zone, grinding temperatures are generally categorized into:
(1) Abrasive grain grinding point temperature
The contact points between the abrasive cutting edge and the workpiece or chips are the areas with the highest temperature during grinding, which can momentarily reach over 1000°C. They not only affect the machined surface quality but also influence abrasive grain wear, fragmentation, chip and abrasive grain adhesion, melting, and wheel clogging.
(2) Grinding zone temperature
This refers to the average temperature in the contact area between the grinding wheel and the workpiece, generally 500-800°C. It affects residual stress, burns, and cracks on the ground surface.
(3) Workpiece average temperature
The grinding heat transferred into the workpiece increases its overall temperature, usually by only a few dozen degrees. It directly affects the shape and dimensional accuracy of the workpiece.
The grinding temperature usually refers to the temperature in the grinding zone. To reduce grinding temperature, one should rationally select grinding parameters, reduce grinding depth, appropriately lower grinding speed, and increase workpiece rotation speed to reduce surface burns and cracks on the workpiece.
Correctly choose the grinding wheel, select coarser abrasive grains, reduce wheel hardness, and dress the wheel in a timely manner. If necessary, use a wheel with large pores; correctly select grinding fluid, increase pressure and flow rate, and pay attention to using appropriate pouring methods to improve cooling and lubrication effects.
IV. Abrasives and Abrasive Tools
1. Conventional abrasives and abrasive tools
Abrasive tools include grinding wheels, grinding stones, mounted points, grinding segments, coated abrasives (sandpaper, sanding belts), and grinding pastes. A grinding wheel is a special cutting tool, also known as an abrasive tool. The structure of an abrasive tool consists of three elements: abrasive grains, bonding agent, and pores, as shown in Figure 7.
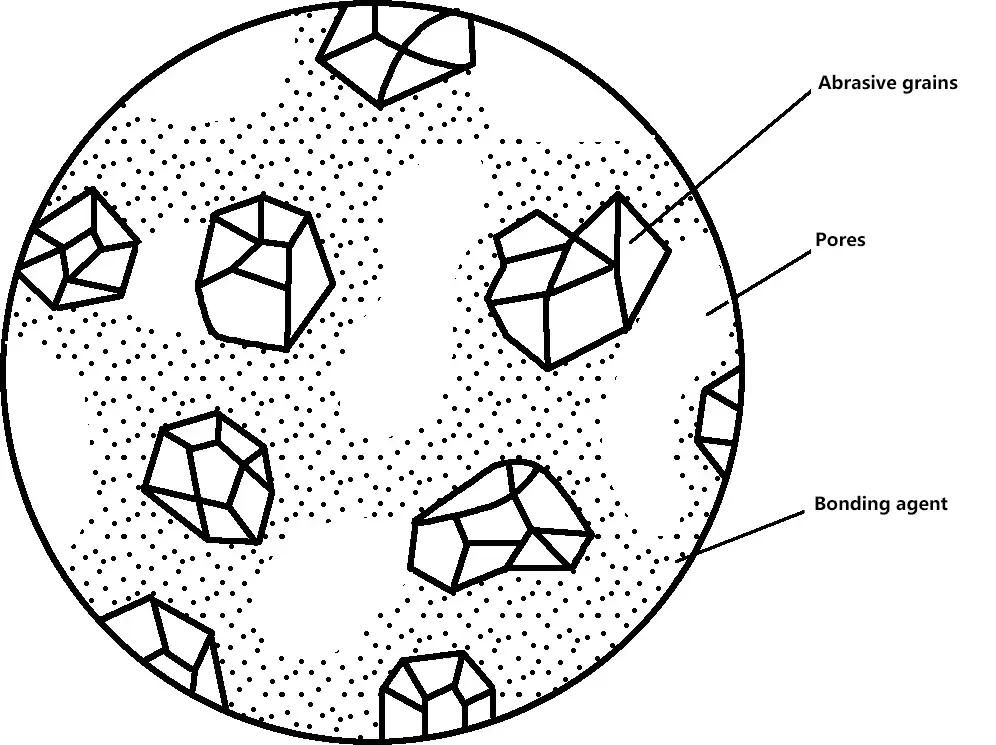
Generally, bonded abrasive tools are made by bonding abrasive grains with a bonding agent through a complex manufacturing process including mixing, molding, drying, sintering, shaping, static balancing, hardness testing, and safety rotation testing. The bonding agent binds the abrasive grains into a specific shape; the exposed parts of the abrasive grains perform the cutting action; the pores accommodate chips, grinding fluid, and help with heat dissipation.
To improve the performance of abrasive tools, certain fillers can be impregnated into the pores, such as sulfur, molybdenum disulfide, wax, resin, etc. Some consider these impregnated substances as the fourth element of bonded abrasive tools.
The working characteristics of abrasive tools are determined by factors such as abrasive material, grain size, bonding agent, hardness, structure, shape and dimensions, strength, and static balance. Different working characteristics have their own applicable ranges, which are briefly introduced below.
(1) Abrasives
Abrasives are the main raw material for manufacturing abrasive tools and directly responsible for the cutting work. To meet the needs of various processing requirements, abrasives should have high hardness, certain toughness, and mechanical strength. Additionally, they should possess thermal stability and chemical stability. Currently, commonly used abrasives include brown aluminum oxide (A), white aluminum oxide (WA), black silicon carbide (C), and green silicon carbide (GC).
Abrasives are divided into two main categories: natural abrasives and artificial abrasives. Natural abrasives generally have disadvantages such as high impurity content and uneven quality, so they are rarely used. Currently, artificial abrasives are mainly used.
Artificial abrasives are further divided into conventional abrasives (including alumina-based and carbide-based types) and superabrasives (including synthetic diamond and cubic boron nitride). The characteristics and application ranges of conventional abrasives are shown in Table 2.
Table 2 Characteristics and application ranges of conventional abrasives
Category | Abrasive name and code | Characteristics | Application range |
Alumina-based | Brown aluminum oxide A(GZ) | Brown color, good toughness, relatively high hardness, inexpensive | Used for grinding materials with relatively low hardness and high plasticity, suitable for grinding medium carbon steel, low carbon steel, low alloy steel, malleable cast iron, hard bronze, etc. Widely applied |
White aluminum oxide WA(GB) | White color, higher hardness than brown aluminum oxide, lower toughness than brown aluminum oxide. Sharp edges, good self-sharpening, lower heat generation during grinding compared to brown aluminum oxide | Used for grinding materials with relatively high hardness and plasticity, suitable for grinding hardened steel, high carbon steel, high-speed steel, threads, gears, and thin-walled parts, etc. | |
Single crystal aluminum oxide SA(GD) | Light yellow or white color, higher hardness and toughness than white aluminum oxide. Spherical particles, good wear resistance, strong cutting ability | Suitable for grinding stainless steel and high vanadium high-speed steel and other materials with high strength and toughness. It can also be used for high-speed grinding and surface grinding requiring low surface roughness | |
Chrome aluminum oxide PA(GG) | Rose or pink color, higher toughness than white aluminum oxide, hardness similar to white aluminum oxide, produces lower surface roughness on ground workpieces | Suitable for edge grinding of hardened steel and alloy steel tools, and precision grinding of threaded parts, measuring tools, and instrument components | |
Microcrystalline aluminum oxide MA(GW) | Color similar to brown aluminum oxide, abrasive grains composed of many small crystals, good toughness, high strength | Suitable for grinding stainless steel, carbon steel, bearing steel, and special nodular cast iron materials. Can also be used for heavy-duty and high-speed grinding | |
Zirconia aluminum oxide ZA(GA) | Grayish-white color, high toughness, good wear resistance | Suitable for heavy-duty grinding, grinding of heat-resistant alloy steels, cobalt alloy steels, and austenitic stainless steels | |
Black aluminum oxide BA(GH) | Black color, lower hardness than brown aluminum oxide, has certain toughness | Suitable for polishing, rust removal, and buffing | |
Praseodymium-neodymium aluminum oxide NA(GP) | Grayish-white color, higher hardness than white aluminum oxide, lower toughness than white aluminum oxide | Suitable for grinding nodular cast iron, high phosphorus cast iron, stainless steel, and super-hard high-speed steel | |
Carbide-based | Black silicon carbide C(TH) | Black with luster, higher hardness than alumina types, low toughness, good thermal conductivity, good self-sharpening, sharp edges | Suitable for grinding brittle materials with low hardness and strength, such as cast iron, bronze, brass, and for grinding, polishing, and cutting of glass, ceramics, leather, rubber, plastics, gemstones, jade, etc. |
Green silicon carbide GC(TL) | Green color, hardness second only to boron carbide and diamond, low toughness, sharp edges, good self-sharpening, relatively expensive | Used for processing high-hardness brittle materials. In addition to the same applications as black silicon carbide, it is mainly used for edge grinding of carbide tools, thread grinding, and is also suitable for cutting and polishing gemstones, jade, precious metals, and semiconductors | |
Boron carbide BC(TP) | Black color, hardness second only to diamond, good wear resistance | Suitable for grinding and polishing tools, abrasive tools, and precision components made of carbide, ceramics, and gemstones | |
Cubic silicon carbide SC | Light green color; cubic crystal structure, higher strength than black silicon carbide, strong grinding ability | Grinding tough and sticky materials such as stainless steel; grinding bearing grooves or superfinishing bearings, etc. |
From Table 2, it can be seen that for grinding materials with higher tensile strength, abrasives with greater toughness from the alumina family should be chosen. For grinding materials with lower tensile strength, abrasives with greater brittleness and higher hardness from the carbide family should be selected. The grades of brown aluminum oxide, white aluminum oxide, and silicon carbide abrasives are shown in Table 3.
Table 3 Grades of brown aluminum oxide, white aluminum oxide, and silicon carbide abrasives
(Reference GB/T2478—2008, GB/T2479—2008, GB/T2480—2008)
Abrasive type | Application | Grade |
Brown aluminum oxide | Ceramic bonded abrasive tools | A |
High-speed sanding belts (including machine-processed sheet sandpaper) | A-P1 | |
Sheet sandpaper | A-P2 | |
Resin and rubber bonded abrasive tools | A-B | |
Sandblasting, polishing | A-S | |
White aluminum oxide | Ceramic bonded abrasive tools | WA |
Organic bonded abrasive tools | WA-B | |
Coated abrasives | WA-P | |
Black silicon carbide | Ceramic bonded abrasive tools, sanding belts | C |
Organic bonded abrasive tools | C-B | |
Manual sheet-type coated abrasives | C-P | |
Green silicon carbide | Ceramic bonded abrasive tools, sanding belts | GC |
Organic bonded abrasive tools | GC-B | |
Manual sheet-type coated abrasives | GC-P |
(2) Grain size
Grain size represents the size of abrasive particles. There are two methods to express grain size: sieve method and photoelectric sedimentation or sedimentation tube granulometer method. The sieve method is expressed by the size of the mesh openings.
Micrograins are measured by sedimentation time. According to GB/T2481.1-1998, coarse abrasive grains are divided into 26 grades from F4 to F220, with smaller numbers indicating coarser grains. According to GB/T 2481.2-2009, micrograins are divided into 13 grades from F230 to F2000, with larger numbers indicating finer grains.
Considerations for grain size selection:
1) When high processing accuracy is required, choose finer grain sizes. Finer grains mean more abrasive particles participate in cutting simultaneously, leaving smaller cutting marks on the working surface, resulting in higher surface quality.
2) When the contact area between the abrasive tool and the workpiece is large or the grinding depth is significant, coarser grain size abrasive tools should be selected. This is because coarser grain tools have less friction with the workpiece and generate less heat. For example, when grinding flat surfaces, using the end face of the grinding wheel requires coarser grains compared to using the circumference.
3) The grain size for rough grinding should be coarser than for fine grinding, which can improve production efficiency.
4) For cutting and groove grinding operations, grinding wheels with coarse grain size, loose structure, and higher hardness should be selected.
5) When grinding soft metals or ductile metals, the wheel surface is easily clogged with chips, so a coarse-grained wheel should be chosen. For grinding high-hardness materials, a relatively coarse grain size should be selected.
6) For form grinding, to better maintain the wheel shape, a finer grain size should be chosen.
7) For high-speed grinding, to improve grinding efficiency, the grain size should be 1-2 grades finer than for normal grinding. This is because with finer grains, there are more abrasive particles per unit working area, and each grain bears less force, making it less prone to dulling. The application range of different grain sizes can be seen in Table 4.
Table 4 Application range of different grain size abrasives
Below F14 | Used for rough grinding or heavy-duty grinding, leather grinding, floor grinding, sandblasting, rust removal, etc. |
F14~F30 | Used for grinding steel ingots, cast iron deburring, cutting steel billets and tubes, rough surface grinding, grinding marble and refractory materials |
F30~F46 | Used for rough grinding of hardened steel parts, brass, and carbides on general surface grinders, cylindrical grinders, centerless grinders, tool grinders, etc. |
F60~F100 | Used for fine grinding, various cutting tool edge grinding, thread grinding, coarse lapping, honing, etc. |
F100~F220 | Used for cutting tool edge grinding, thread grinding, fine grinding, coarse lapping, honing, etc. |
F150~F1000 | Used for fine grinding, thread grinding, gear fine grinding, precision instrument parts fine grinding, fine lapping and honing, etc. |
Above F1000 | Used for super-fine grinding, mirror grinding, fine lapping and polishing, etc. |
(3) Bonding agent
The main function of the bonding agent is to bind the abrasive grains together to form abrasives with specific shapes and strengths. The codes, properties, and application ranges of commonly used bonding agents are shown in Table 5.
Table 5 Codes, properties, and application ranges of common abrasive bonding agents
Name and code | Properties | Application range |
Vitrified bond V(A) | Chemically stable, heat-resistant, acid and alkali resistant, high porosity, low wear, high strength, good shape retention, widely used Boron-containing vitrified bond, high strength, less bonding agent required, can correspondingly increase the porosity of the abrasive | Suitable for internal grinding, external grinding, centerless grinding, surface grinding, form grinding and thread grinding, tool sharpening, honing and super-finishing. Suitable for grinding various steels, cast iron, non-ferrous metals, glass, ceramics, etc. Suitable for high-porosity grinding wheels |
Resin bond B(S) | High bonding strength, has some elasticity, easily burned at high temperatures, good self-sharpening, good polishing properties, not resistant to acids and alkalis Can add graphite or copper powder to make conductive grinding wheels | Suitable for rough grinding, cutting and free grinding, such as thin disc wheels, high-speed, heavy-duty, low surface roughness grinding, deburring of castings and forgings, and conductive grinding wheels |
Reinforced resin bond BF | Resin bond with added glass fiber mesh to increase wheel strength | Suitable for high-speed wheels (vs=60~80m/s), thin disc wheels, grinding welds or cutting |
Rubber bond B(S) | High strength, more elastic than resin bond, lower porosity, abrasive grains easily fall off after dulling. Disadvantages include poor heat resistance (150°C), not resistant to acids and alkalis, and odor during grinding | Suitable for fine grinding, mirror grinding wheels, ultra-thin disc wheels, polishing wheels for bearings, blades, drill bit grooves, etc., and guide wheels for centerless grinding |
Magnesite bond Mg(L) | Lower bonding strength than vitrified bond, but has good self-sharpening properties, generates less heat during operation, thus sometimes providing better grinding effects in certain processes than other bonding agents. Disadvantage is that it’s easily hydrolyzed and not suitable for wet grinding | Suitable for grinding materials with poor heat conductivity and grinding with large contact areas between the abrasive and workpiece Suitable for safety razor blades, paper cutting knives, agricultural tools, grain processing, floor and colloidal material processing, etc. Wheel speed is generally less than 20m/s |
(4) Hardness
The hardness of an abrasive refers to the degree of difficulty with which the abrasive grains on the surface of the abrasive detach from the bonding agent under the action of cutting forces. If the grains detach easily, the abrasive has low hardness, and vice versa. It’s important not to confuse the hardness of the abrasive with the hardness of the abrasive grains themselves.
The main factor affecting abrasive hardness is the amount of bonding agent; more bonding agent results in higher abrasive hardness. Additionally, in the abrasive manufacturing process, the forming density, firing temperature, and duration all affect the abrasive’s hardness.
The basic principle for selecting abrasive hardness is to ensure appropriate self-sharpening of the abrasive during the grinding process, avoid excessive abrasive wear, and ensure that excessive grinding temperatures are not produced during grinding.
1) When the workpiece hardness is higher, the abrasive hardness should be lower, and vice versa. This is because when the workpiece is hard, the abrasive grains bear higher pressure during grinding and are more likely to become dull. Choosing a softer wheel can promote timely self-sharpening and maintain the wheel’s grinding performance.
When the workpiece hardness is lower, the abrasive grains dull more slowly. To prevent the grains from detaching before they become dull, a harder wheel should be chosen. However, when the workpiece has low hardness but high toughness, chips can easily clog the wheel, so a wheel with coarser grains and lower hardness should be selected.
2) Generally, harder wheels are chosen for rough grinding compared to fine grinding; for internal grinding, as the contact area between the wheel and workpiece is larger than in external grinding, which can cause workpiece heating, a softer wheel should be chosen. However, when the internal hole diameter is small, the wheel speed is lower, and the wheel has good self-sharpening properties, a slightly harder wheel can be selected; for high-speed grinding, as the wheel has poor self-sharpening properties, the wheel hardness should be 1-2 grades lower.
3) For form grinding, to maintain the wheel shape, a harder wheel should be chosen; when grinding discontinuous surfaces, as the abrasive grains are prone to detachment due to impact, a harder wheel can be selected.
4) For materials with poor heat conductivity that are prone to burning (such as high-speed steel tools, bearings, thin-walled parts, etc.), softer wheels should be chosen.
5) When the contact area between the wheel and workpiece is large, a softer wheel should be chosen. For example, when using the end face of a wheel for surface grinding, it should be softer than when using the outer diameter of the wheel.
6) For fine grinding, where high surface quality is required, a softer wheel should be chosen; for low surface roughness grinding, ultra-soft wheels are often selected. The hardness range selection for different grinding methods can be referenced in Table 6.
Table 6 Hardness range selection for different grinding methods
1 | 1—Regular cylindrical grinding | |||||||||||||
2 | 2—Centerless cylindrical grinding | |||||||||||||
3 | 3—Roll grinding | |||||||||||||
4 | 4—Surface (horizontal spindle) grinding | |||||||||||||
5 | 5—Surface (vertical spindle) grinding | |||||||||||||
6 | 6—Internal grinding | |||||||||||||
7 | 7—Tool grinding | |||||||||||||
8 | 8—Thread grinding | |||||||||||||
9 | 9—Low surface roughness grinding | |||||||||||||
1 | 10—Heavy-duty grinding | |||||||||||||
1 | 11—Honing | |||||||||||||
1 | 12—Creep feed grinding | |||||||||||||
1 | 13—Deburring grinding | |||||||||||||
FGHJKLMNPQRSTY | ||||||||||||||
Soft ↔ Hard |
2. Superabrasive tools
Superabrasives refer to diamond and cubic boron nitride abrasives. Diamonds include natural diamonds and synthetic diamonds. Natural diamond is the hardest substance in nature, with low production and high price, so synthetic diamonds are generally used more often.
Cubic boron nitride is boron nitride with a cubic crystal structure, with the molecular formula BN. Its crystal structure is similar to diamond, with a hardness slightly lower than diamond, ranging from 72000 to 98000 HV. To date, no natural cubic boron nitride has been discovered, and it is currently produced artificially.
(1) Characteristics of synthetic diamond abrasives
- Can process various high-hardness, high-brittleness materials such as carbides, ceramics, optical glass, semiconductor materials, etc.
- Diamond abrasive grains have sharp edges, strong grinding ability, long life, and small grinding force, only 1/4 to 1/5 of green silicon carbide, which is beneficial for improving workpiece accuracy and reducing surface roughness.
- Diamond grinding wheels have low grinding temperatures, which can prevent surface burning, cracking, and structural changes in the workpiece.
- Diamond grinding wheels have long life, low wear, save working hours, and are economical to use.
- Relatively low heat resistance (700~800°C), losing cutting ability at high cutting temperatures. Additionally, diamond has a strong affinity for iron elements, causing chemical wear, and is generally not suitable for grinding ferrous materials.
(2) Characteristics of cubic boron nitride (CBN) abrasives
Besides being used to make cutting tools, the largest application area of CBN materials is in the production of CBN abrasives, used for high-speed and high-efficiency grinding and honing processes. This can greatly improve grinding efficiency, and enhance grinding precision and quality by one level.
1) Good thermal stability.
Its heat resistance temperature is above 1200°C, with strong chemical inertness, and it does not easily react chemically with ferrous elements. Therefore, it is suitable for processing hard and tough steel parts (such as super-hard high-speed steel), as well as materials with high hardness and low thermal conductivity at high temperatures.
2) Good wear resistance.
For example, when grinding alloy tool steel, its lifespan is more than 100 times higher than ordinary corundum wheels, which is conducive to achieving automated processing.
3) High production efficiency.
When processing materials such as hard alloys, diamond wheels are superior to cubic boron nitride wheels. However, when processing alloy steels like high-speed steel, heat-resistant steel, and abrasive steel, its metal removal rate is 10 times that of diamond wheels and 60-100 times that of white corundum wheels.
4) Good grinding quality.
The dimensional accuracy of the ground workpiece is high, the surface roughness value is low, and it is not prone to burns and cracks. The surface residual stress is smaller than that of corundum wheels.
5) Low processing cost.
Although cubic boron nitride wheels are expensive, they have advantages such as high processing efficiency, good surface quality, long lifespan, easy control of dimensional accuracy, and low scrap rate, resulting in lower overall costs.
The common characteristics of the two super-hard abrasives are high hardness, good thermal conductivity, and sharp edges, but each has its own characteristics. Diamond has poor thermal stability and will diffuse to form carbides with metals such as iron, chromium, vanadium, tungsten, molybdenum, and titanium at high temperatures, accelerating abrasive wear. Therefore, it is not suitable for grinding ferrous metals but can grind various alloy cast irons, as the carbon in cast iron is saturated and no further diffusion occurs.
For example, when grinding aluminum-silicon alloy die-cast parts, higher processing surface quality and economic benefits can be achieved. The lifespan of diamond abrasives can be increased by about 40% with wet grinding compared to dry grinding, so wet grinding should be used as much as possible.
Cubic boron nitride is suitable for grinding ferrous materials and is commonly used for grinding crankshafts, camshafts, internal holes, gears, difficult-to-machine materials, and surfaces. It can achieve high-speed grinding and high feed rate grinding, resulting in low surface roughness values without burning, and can obtain higher precision while improving grinding efficiency.
CBN wheels have a long lifespan and good grinding performance, saving auxiliary time for wheel replacement, dressing, machine adjustment, and workpiece inspection. During grinding, extreme pressure emulsions or high-speed grinding fluids are generally used for cooling. The comparison of the application ranges of the two super-hard abrasives is shown in Table 7.
Table 7 Application ranges of two super-hard abrasives
Workpiece material | Cubic boron nitride | Diamond | ||
Wet grinding | Dry grinding | Wet grinding | Dry grinding | |
Various high-speed steels | √ | √ | × | × |
Alloy tool steel | √ | √ | × | × |
Stainless steel, heat-resistant steel | √ | √ | √ | × |
Chrome steel | √ | √ | × | × |
Abrasive steel | × | √ | √ | × |
Cast iron | × | √ | √ | × |
Carbide | × | × | √ | √ |
Glass, ceramics, semiconductors | × | × | √ | √ |
Non-ferrous metals | × | × | √ | √ |
Note: √—Applicable, ×—Not applicable.
3. Coated abrasives
Coated abrasives are abrasives made by adhering abrasive grains to a flexible backing material with adhesives, commonly known as “sandpaper and sandcloth”. Currently, these products in China mainly include: sandcloth, sandpaper, sanding belts, sanding discs, flap wheels, and sanding sleeves.
The characteristics of coated abrasives are easy to use, simple equipment, safe operation; short production cycle of abrasives, and low price. With the introduction of paper sanding belts and steel paper grinding discs, the application range of coated abrasives has become even wider.
(1) Classification and characteristics of coated abrasives
1) Classification of coated abrasives
The classification of coated abrasives is shown in Table 8.
Table 8 Classification of coated abrasives
Name | Type |
Sandcloth | Waterproof sandcloth |
Flap wheel | |
Roll sandcloth | |
Sandpaper | Waterproof sandpaper |
Sheet sandpaper | |
Roll sandpaper | |
Metallographic sandpaper | |
Sanding belt | Waterproof sanding belt |
Cloth sanding belt | |
Waterproof paper sanding belt | |
Paper sanding belt | |
Composite backing sanding belt | |
Seamless sanding belt | |
Jointed sanding belt | |
Overlap sanding belt | |
Symmetrical sanding belt | |
Sanding disc | Bonded sanding disc |
Sanding sleeve | Cylindrical sanding sleeve |
Conical sanding sleeve | |
Flap wheel | Shafted flap wheel |
Chuck flap wheel |
2) Grit size of coated abrasives
GB/9258.2—2008 specifies the grit size of coarse abrasive grains for coated abrasives, and GB/T9258.3—2000 specifies the grit size of abrasive micrograins for coated abrasives.
The grit size of coarse abrasive grains is divided into 20 grit numbers: P12, P14, P16, P20, P24, P30, P36, P40, P50, P60, P70, P80, P100, P120, P150, P180, P220.
The grit size of fine abrasive powders is divided into 13 grit numbers: P240, P280, P320, P360, P400, P500, P600, P800, P1000, P1200, P1500, P2000, P2500.
3) Adhesives for coated abrasives
The adhesives for coated abrasives are mainly liquid materials used to bond abrasive grains and backing materials. The types and codes of adhesives for coated abrasives are shown in Table 9; the performance and application range of various adhesives for coated abrasives are shown in Table 10.
Table 9 Types of adhesives and their codes
Name | Animal glue adhesive | Semi-resin adhesive | Full resin adhesive | Waterproof adhesive |
Code (base coat/size coat) | G/G | R/G | R/R | WP |
Table 10 Performance and application range of various adhesives for coated abrasives
Category | Binder Name | Performance | Applicable Range |
Animal Glue G/G | Hide Glue | Good adhesion performance, low strength, soluble in water, easily affected by moisture, stability influenced by environment, poor heat resistance, brittle, but inexpensive | Can be used for dry and oil grinding with low cutting force, suitable for sanding wood products, processing non-metallic products, grinding and polishing of copper, lead, and other metals |
Gelatin | |||
Bone Glue | |||
Full Resin R/R | Alkyd Resin | Higher adhesion strength, water and heat resistant, can be stored for a longer time, suitable for dry and wet grinding, but soluble in organic solutions, higher cost | Suitable for heavy-duty grinding, difficult-to-grind materials, and complex surface metal forming grinding and polishing |
Amino Resin | |||
Waterproof Sandpaper Varnish | |||
Semi-resin R/C | Urea-formaldehyde Resin Hide Glue Gelatin | Base glue is animal glue, with good elasticity, wear resistance, and heat resistance, not water-resistant but moisture-resistant, better adhesion performance than animal glue, easy to manufacture, lower cost, generally used for dry grinding | Suitable for dry or oil grinding, medium-load grinding, and forming surface processing. This type of binder has been widely used in sanding belt grinding |
4) Backing Material
Backing material refers to the carrier of abrasives and binders in coated abrasives, generally using soft fibrous materials, requiring high tensile strength, low elongation, and good water resistance. The backing materials and uses of coated abrasives are shown in Table 11; backing material classifications and codes are shown in Table 12.
Table 11 Backing Materials and Uses of Coated Abrasives
Backing Material Category | Material | Uses |
Cloth Backing | Coarse and fine twill, plain weave, and canvas, etc. | Dry sanding, waterproof sandpaper, sanding belts, etc. |
Paper Backing | Kraft paper, waterproof kraft paper, vulcanized fiber paper, etc. | Dry sanding, waterproof sandpaper, vulcanized fiber discs, etc. |
Vulcanized Fiber Backing | Backing made of vulcanized fiber paper. Note: Vulcanized fiber paper is a homogeneous material made by treating cellulose with concentrated sulfate or zinc oxide to change the physical properties of the fibers | Sanding belts, high-speed vulcanized fiber paper, grinding discs, etc. |
Composite Backing | Backing made of cloth and paper composites, with mesh cloth or vulcanized fiber paper between two layers of paper | Sanding belts |
Table 12 Backing Material Classifications and Codes
Type | Cloth-backed Abrasive | Sandpaper | Waterproof Sandpaper | ||||||||||
Light Cloth | Medium Cloth | Heavy Cloth | Sheet S | Roll R | Sheet S | Roll R | |||||||
Surface Density/g·m-2 | ≥110 | ≥170 | ≥250 | 80 | 100 | 120 | 160 | 220 | 80 | 100 | 125 | 160 | |
Code | L | M | H | A | B | C | D | E | A | B | C | D |
(2) Cloth-backed Abrasives, Sandpaper Discs, Flap Wheels, and Sanding Sleeves
1) Dry Sanding Cloth
Dry sanding cloth is generally used for manual or low-speed, low-pressure dry sanding. When using oil-based cutting fluids, efficiency and processing quality can be improved. After exposure to moisture, dry sanding cloth can be slightly dried at a temperature of 60-80°C; excessive drying may lead to breakage.
Dry sanding cloth is generally produced in sheet form (code S) and roll form (code R); based on surface density, it is divided into light (L), medium (M), and heavy (H) types. Light cloth is suitable for wood, leather, rubber, and other processing, while heavy cloth is suitable for metal processing.
2) Waterproof Sandpaper
It should be soaked in 40°C water for about 10 minutes before use, and used after softening. Grit selection: P80-P150 for coarse grinding, P180-P280 for fine grinding, and P320 and above for precision grinding. The two most commonly used abrasives for waterproof sandpaper are glass sand (code GL) and garnet (code G).
Marking: Sandpaper S G/G C230×280 A P80
3) Vulcanized Fiber Discs
Vulcanized fiber discs are made by adhering abrasives to the surface of high-quality vulcanized fiber paper using high-strength heat-resistant synthetic resin as a binder, forming circular thin discs. Vulcanized fiber discs can be mounted on handheld machines for grinding and polishing, used for rust removal, polishing, and other dry grinding processes on metal or non-metal materials. They are easy to operate, highly efficient, versatile, and safe and reliable. The maximum working speed of vulcanized fiber discs is 80m/s.
4) Abrasive Flap Wheels
Flap wheels are wheel-shaped or disc-shaped abrasive tools formed by evenly distributing sheet-like cloth-backed abrasives radially from the axis.
Abrasive flap wheels have relatively high processing efficiency and are widely used for grinding and polishing. They are effective for polishing non-ferrous metals and stainless steel products, as well as for finishing shaped surfaces of abrasive tools. End face flap wheels are suitable for large area polishing and finishing. Flap wheels are convenient to use and can be used with electric and pneumatic tools, as well as lathes, drilling machines, and other machine tools.
Maximum working speed for flap wheels: 35m/s or 50m/s for shaft-mounted and chuck-mounted flap wheels, 80m/s for cup-shaped flap wheels.
5) Cylindrical Sanding Sleeves
Cylindrical sanding sleeves, also known as cloth-backed sanding sleeves (code S), are cylindrical abrasive tools made by adhering abrasives to cloth or paper backing material. Their uses are similar to flap wheels and are more suitable for polishing large bearings, with higher efficiency. Sanding sleeves are divided into cylindrical cloth-backed sanding sleeves (code SC) and cylindrical paper-backed sanding sleeves (code SP).
(3) Sanding Belts
Sanding belts are loop-shaped coated abrasives, divided into endless belts and jointed belts. Endless belts have been gradually replaced by jointed belts due to limitations in backing material manufacturing processes and low production rates. Currently, in domestic and international sanding belt standards, sanding belts actually refer to jointed belts.
Sanding belt grinding is a new type of grinding process that has developed over 30 years into a relatively complete and self-contained processing technology. Sanding belts are the second generation of coated abrasive products. Sanding belt grinding is a highly efficient continuous processing method performed on specific equipment, with a wide range of applications, hence called “universal grinding” technology.
1) Classification of Sanding Belts
There are many types of sanding belts. By shape, there are roll belts and loop belts; by backing material, there are cloth belts, paper belts, and composite backing belts; by binder, there are animal glue belts, semi-resin belts, and full resin belts; by working surface, there are dense-grain belts, sparse-grain belts, and super-coated belts. The types and characteristics of sanding belts are shown in Table 13.
Table 13 Types and Characteristics of Sanding Belts
Belt Type | Backing Material | Tensile Strength/kPa | Elongation at 600N Load (%) |
Cloth Belt | H Cloth | ≥32 | ≤3.0 |
M Cloth | ≥20 | ≤4.5 | |
L Cloth | ≥15 | ≤5.0 | |
Paper Belt | C Type Paper | ≥8.4 | — |
E Type Paper | ≥24 | ≤1.5 | |
Multi-joint Belt | E Type Paper | ≥8 | — |
Composite Backing Belt | Composite Backing | ≥32 | ≤1.0 |
Multi-joint Composite Backing Belt | Composite Backing | ≥13 | ≤3.0 |
2) Selection of sanding belt grit
The selection of sanding belt grit is mainly based on processing conditions and surface roughness requirements of the workpiece. Its applicable range and achievable surface roughness are shown in Table 14.
Table 14 Applicable range and achievable surface roughness of sanding belt grit
Grit size | Applicable processing range | Achievable surface roughness Ra/μm |
P16~P24 | Rough grinding of cast iron, welded parts, and deburring, etc. | — |
P30~P40 | Rough grinding of internal circles, external circles, planes, and curved surfaces | >32 |
P50~P120 | Semi-finish and finish grinding of internal circles, external circles, planes, and curved surfaces | 1.6~0.8 |
P150~P240 | Fine grinding, profile grinding | 0.8~0.2 |
P280~P1200 | Fine grinding, super-fine grinding, and mirror finishing, etc. | Below 0.2 |
3) Selection of sanding belt adhesive
The types, properties, and applicable ranges of sanding belt adhesives are shown in Tables 9 and 10.
In addition to this, some sanding belts have special requirements, with an extra layer of super-coating adhesive applied over the resin layer.
① Antistatic coating adhesive.
It is mainly used for processing wood and plastic products, which can prevent the adhesion of cutting dust caused by static electricity generated from friction between the back of the sanding belt and the support, which is beneficial for production safety.
② Anti-clogging super-coating adhesive.
It is a resin coating mainly composed of metal soap, which can effectively prevent clogging of the sanding belt surface, thereby improving grinding efficiency and belt life.
③ Anti-oxidation decomposition super-coating adhesive.
It is composed of elastic polymer materials and active materials resistant to oxidation and decomposition. It has a cooling effect during processing, which can effectively improve the life of the sanding belt and the quality of the processed surface.
4) Selection of sanding belt backing material
The selection of sanding belt backing material is mainly based on usage requirements. For high processing loads and high speeds, the backing material requires higher strength and lower elongation. Generally, cotton and linen fabrics or nylon and polyester fiber fabrics are chosen as backing materials. Common sanding belts often use different weight grades of textiles and paper materials based on the fabric’s areal density and different grit sizes.
Paper-backed sanding belts are smoother and more even than cloth-backed ones, but they have lower load-bearing capacity. Composite backing materials combine the advantages of both and are suitable for heavy-duty grinding. There are two types of composite backing materials: one is to add a layer of mesh cloth between two layers of paper; the other is to add a layer of fabric on top of the paper backing.