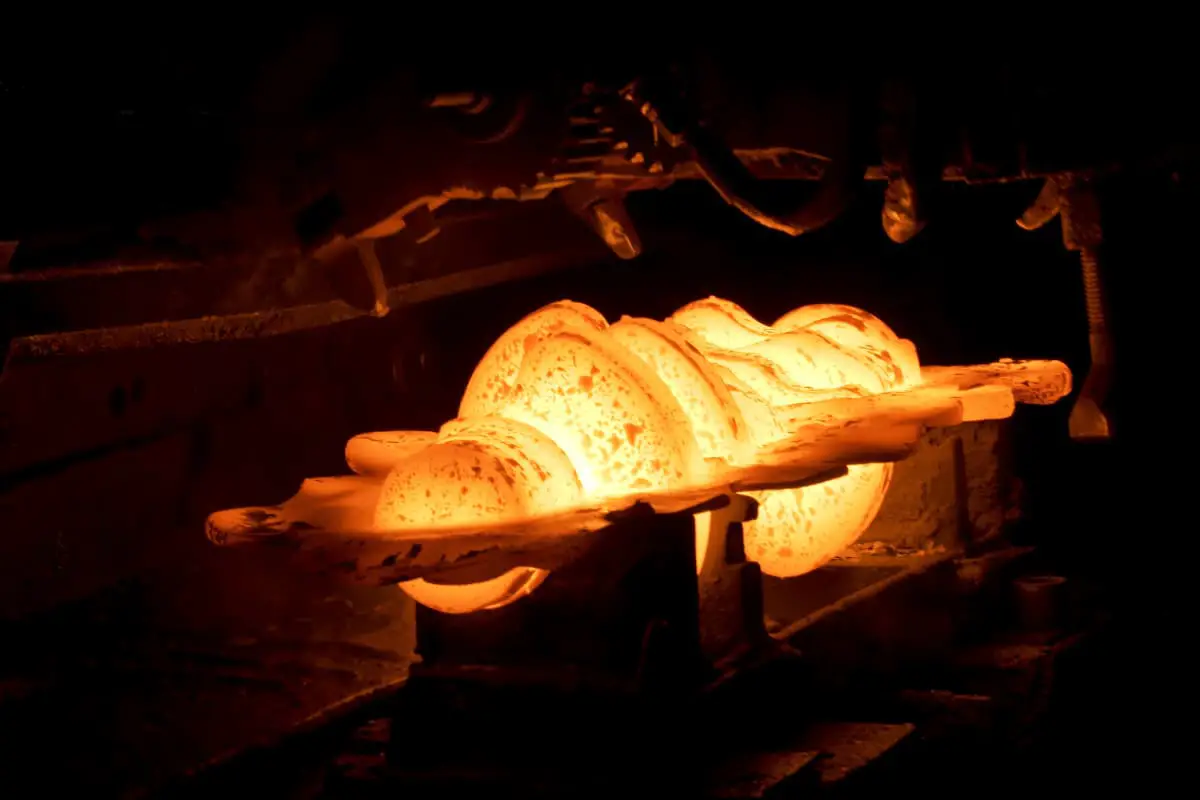
Annealing and Normalizing of Steel
Annealing and normalizing are widely applied heat treatment processes in manufacturing, primarily used for the preparatory heat treatment of castings, forgings, and welded components.
The goal is to eliminate thermal processing defects, improve microstructure, and enhance machinability. For parts with less stringent performance requirements, these processes can also serve as the final heat treatment. The heating temperature ranges for various annealing and normalizing processes are illustrated in Figure 1-37.
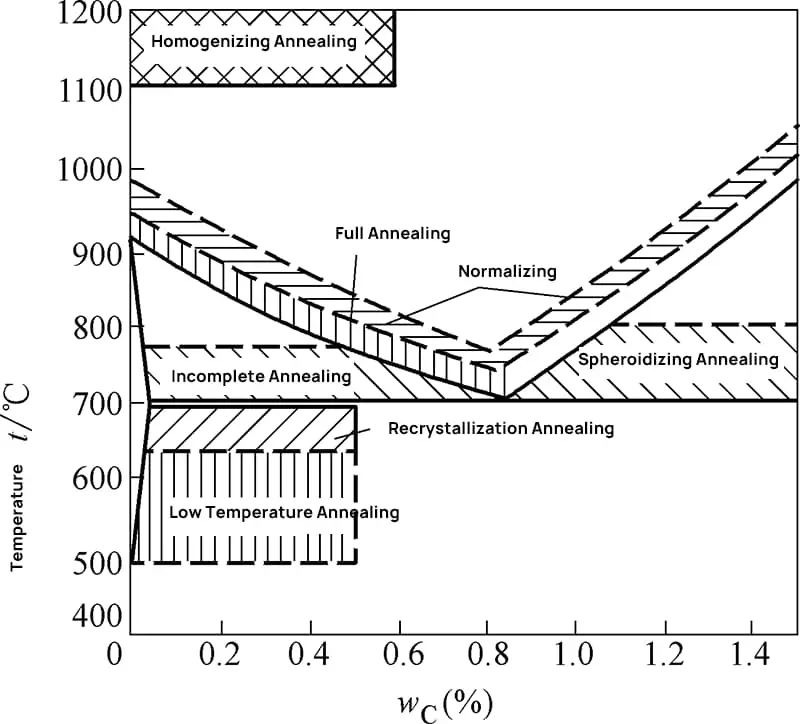
Annealing
Annealing involves heating steel to a temperature either above or below the critical transformation point, then maintaining the temperature followed by slow cooling in the furnace to obtain a near-equilibrium microstructure. There are many types of annealing processes; the ones commonly used in production include:
1) Full Annealing.
Full annealing heats the steel component to 30-50°C above the Ac1 temperature, holds it for a sufficient time to fully austenitize the structure, then cools slowly to achieve a near-equilibrium microstructure.
This process is mainly for hypoeutectoid steels with higher carbon content, aiming to refine grains, homogenize the microstructure, relieve internal stresses, reduce hardness, and improve machinability. The resultant microstructure consists of ferrite plus pearlite.
2) Incomplete Annealing.
Incomplete annealing heats steel to a temperature between Ac1 and Ac3 for hypoeutectoid steels or between Ac1 and Ac for hypereutectoid steels, followed by slow cooling after holding to achieve a near-equilibrium microstructure. Since the steel is heated to the two-phase region, the morphology and distribution of proeutectoid ferrite or secondary cementite are essentially unchanged.
Incomplete annealing is primarily used for hypereutectoid steels to obtain a spheroidized pearlite structure, hence it is also known as spheroidizing annealing for hypereutectoid steels. The purpose is to transform the microstructure, converting network cementite into a spheroidal form, relieving internal stresses, reducing hardness, improving machinability, and preparing the structure for quenching.
3) Stress-Relief Annealing.
Stress-relief annealing heats the steel component to a temperature below Ac1, maintains the temperature, then cools slowly. No phase transformation occurs during this process; its purpose is to eliminate residual internal stresses in castings, forgings, welded components, and machined parts, enhancing dimensional stability and preventing deformation and cracking.
Due to the lower heating temperature, it is also known as subcritical annealing.
4) Recrystallization Annealing.
Recrystallization annealing heats cold-worked metal above the recrystallization temperature, holds it for an appropriate time, allowing the deformed grains to transform back into uniform, equiaxed grains while eliminating work hardening and residual stresses.
This process can be used as an intermediate annealing between multiple cold workings of a metal or as the final heat treatment of cold-worked metal. After recrystallization annealing, the metal’s microstructure and properties are restored to their pre-cold-worked state.
Annealing
Normalizing is a heat treatment process that heats steel parts to 30-50°C above the Ac3 or Acm temperature, holds them for an appropriate time, and then cools them in air to obtain a pearlitic structure. The normalizing temperature for hypoeutectoid steel is slightly higher than that of full annealing, but it cools faster, resulting in a finer pearlitic structure and higher strength and hardness of the steel.
Normalizing offers higher productivity without occupying equipment for extended periods and is often used to improve the machinability of low-carbon steels. For parts with less stress and lower performance requirements, normalizing can serve as the final heat treatment process. It eliminates the network carbides in hypereutectoid steel, preparing the structure for spheroidizing annealing.
For large workpieces, or those with complex shapes or significant changes in cross-sectional dimensions, normalizing can replace quenching and tempering to prevent distortion and cracking.
Quenching and Tempering of Steel
(1) Quenching
Quenching is a heat treatment process that involves heating steel to a critical phase transition point, Ac3 or above Ac1 by 30-50°C, followed by insulation and rapid cooling to obtain non-equilibrium structures such as martensite and bainite.
The primary purpose of quenching steel is to maximize the formation of martensite, followed by tempering at various temperatures to achieve the desired properties. The quality of quenching depends on the quenching heating temperature, the cooling medium, and the quenching method.
1) Quenching Heating Temperature.
The selection of the quenching heating temperature should be based on obtaining a uniform and fine austenitic grain size, which facilitates the formation of fine martensite structures upon quenching. The specific quenching heating temperature is determined based on the steel’s critical phase transition point.
For hypo-eutectoid steel, the quenching heating temperature is usually above Ac3 by 30-50°C. If the temperature is between Ac1 and Ac3, ferrite will remain in the structure along with martensite after quenching, reducing the steel’s strength and hardness. However, the heating temperature should not exceed Ac3 by too much to prevent austenitic grain coarsening and the formation of coarse martensitic structures.
For eutectoid and hyper-eutectoid steels, the quenching heating temperature is typically above Ac1 by 30-50°C. This is mainly to obtain fine austenitic grains while retaining an appropriate amount of cementite, which results in the formation of cryptocrystalline martensite and evenly distributed granular carbides after quenching.
Consequently, the steel not only possesses higher strength, hardness, and wear resistance but also exhibits good toughness. If the heating temperature is too high, a large number of secondary cementite particles dissolve, increasing the amount of retained austenite after quenching, which can lead to greater deformation and cracking tendencies.
The quenching heating temperature for alloy steels is generally higher than that for carbon steels because most alloying elements hinder the growth of austenitic grains. Raising the quenching heating temperature allows for the full dissolution and homogenization of the alloying elements, leading to better quenching results.
2) Quenching Cooling Medium.
The cooling medium used to bring steel from the austenitic state to below the Ms point is called the quenching cooling medium.
The stronger the cooling capacity of the medium, the greater the cooling rate of the steel, resulting in a deeper hardened layer after quenching. However, too high a cooling rate can induce significant quenching stresses in the workpiece, making it prone to deformation and cracking. Therefore, selecting an appropriate quenching cooling medium is crucial during quenching.
Common quenching coolants include water, brine, alkaline water solutions, and oils, with their cooling capacities presented in Table 1-4. Water and oil are the most frequently used quenching mediums. Typically, water quenching is employed for carbon steel workpieces that are small in size and simple in shape, whereas oil quenching is generally reserved for larger, more complex carbon steel and alloy steel workpieces.
Table 1-4: Cooling Capacity of Commonly Used Quenching Media
Quenching medium | Cooling capacity within the following temperature range (°C/s) | |
650~550℃ | 300~200℃ | |
Water (at 18°C) | 600 | 270 |
10% NaCl aqueous solution (at 18°C) | 1100 | 300 |
10% NaOH aqueous solution (at 18°C) | 1200 | 300 |
10% Na2CO3 aqueous solution (at 18°C) | 800 | 270 |
Mineral oil | 150 | 30 |
Canola oil | 200 | 35 |
Nitrate-based molten salt (at 200°C) | 350 | 10 |
3) Quenching Methods
The common quenching methods include single-medium quenching, double-medium quenching, step quenching, and isothermal quenching, with their cooling curves illustrated in Figure 1-38.
Single-medium quenching involves immersing a workpiece heated to the austenitic state into a quenching medium and continuously cooling it to room temperature (Curve 1 in Figure 1-38). This method is suitable for simple-shaped carbon steel and alloy steel workpieces, generally using water for carbon steels and oil for alloy steels.
Double-medium quenching consists of initially cooling a workpiece heated to the austenitic state in a quenching medium with a strong cooling capacity until it reaches near the Ms point temperature, then immediately transferring it to a medium with a weaker cooling capacity until the martensitic transformation is complete (Curve 2 in Figure 1-38).
This method is typically used for larger carbon steel pieces, often employing water quench followed by oil cooling or oil quench followed by air cooling. Step quenching involves immersing a workpiece heated to the austenitic state into a slightly above Ms point temperature medium (like a salt bath) to equalize internal and external temperatures, then air cooling it to room temperature to complete the martensitic transformation (Curve 3 in Figure 1-38).
This quenching method is applicable to smaller workpieces, such as cutting tools, measuring tools, and precision parts requiring minimal deformation.
Isothermal quenching is the process of immersing a workpiece heated to the austenitic state in a salt bath at an appropriate temperature above the Ms point, holding it for an extended period to transform into lower bainite, then air cooling to room temperature (Curve 4 in Figure 1-38).
This method is mainly used for complex shapes and precision-sized tools and critical machine parts, such as molds, cutting tools, gears, etc.
4) Hardenability of Steel.
Hardenability refers to the ability of austenitized steel to form martensite during quenching, characterized by the depth of the hardened layer and the distribution of hardness obtained under certain conditions. Hardenability is a crucial technological property of steel and is one of the essential criteria for material selection and the formulation of heat treatment processes.
For larger cross-section workpieces, the surface cooling rate is the highest, and the core cooling rate is the lowest after heating and quenching. Across the workpiece’s cross-section, areas cooling faster than the critical cooling rate (v_critical) will fully transform into martensitic structures, while areas with cooling rates below v_critical will form non-martensitic structures, as shown in Figure 1-39.
The depth from the workpiece’s surface to the half-martensite zone (where martensite and non-martensite structures each make up 50% of the area) is typically defined as the depth of the hardened layer. The position of the half-martensite zone can be easily observed with a metallographic microscope and measured for hardness with a durometer.
Therefore, hardenability can also be understood as the steel’s capacity to achieve a certain depth of the hardened layer after quenching, essentially reflecting the stability of the supercooled austenite.
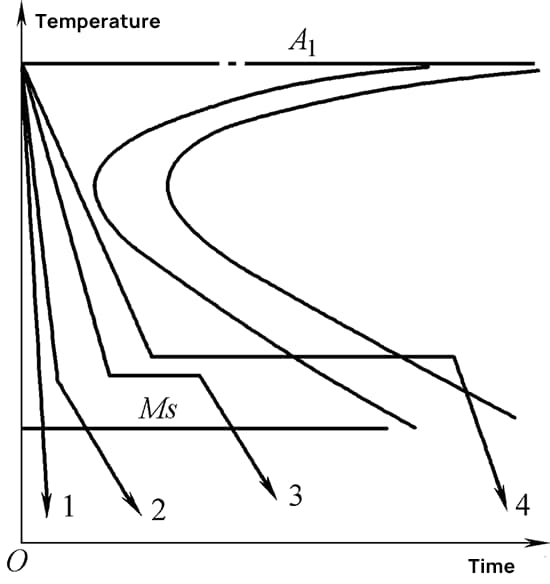
(2) Tempering
Tempering is the process of heating quenched steel to a temperature below critical point A, transforming it into a stable tempered structure, and then cooling it down to room temperature in a controlled manner.
The primary purpose of tempering is to reduce or eliminate quenching stresses, ensure the corresponding structural transformation, enhance the steel’s toughness and plasticity, and achieve a proper balance between strength, hardness, plasticity, and toughness to meet the performance requirements of workpieces for various applications.
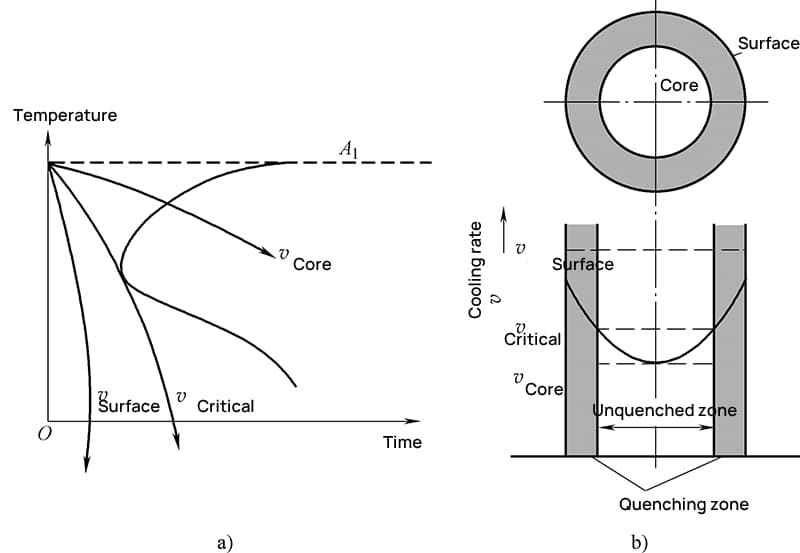
a) Cooling Rate of the Workpiece Cross-Section b) Unhardened Zone
Tempering can be categorized into low, medium, and high-temperature processes, depending on the heating temperatures involved. Low-temperature tempering occurs at 150-250°C and primarily results in tempered martensite. This treatment maintains the steel’s high strength, hardness, and wear resistance while also enhancing toughness. T
herefore, low-temperature tempering is particularly suitable for tools, measuring instruments, rolling bearings, carburized parts, and surface-hardened workpieces. For high-carbon and high-carbon alloy steels, it preserves high hardness and wear resistance while significantly reducing quenching stress and brittleness.
For steels that have achieved low-carbon martensite through quenching, low-temperature tempering can reduce internal stresses and further improve strength and plasticity, maintaining excellent comprehensive mechanical properties.
Medium-temperature tempering is performed at 350-500°C and mainly results in tempered troostite. This process effectively eliminates quenching stress, so the steel exhibits a high elastic limit, and retains high strength and hardness, as well as good plasticity and toughness. Thus, medium-temperature tempering is mainly used for various spring components and hot-work dies.
High-temperature tempering occurs at 500-650°C, leading to tempered sorbite. The heat treatment process that combines quenching and high-temperature tempering is known as quenching and tempering.
After this treatment, the steel possesses excellent comprehensive mechanical properties. Consequently, high-temperature tempering is mainly suitable for important machine parts made of medium-carbon structural steel or low-alloy structural steel, such as shafts, gears, connecting rods, and bolts. These parts require high strength and the ability to withstand impact and alternating loads during use.