When investing in a laser cutting machine for processing services, it’s crucial to understand that accurate quotations stem from a comprehensive analysis of laser cutting costs. The final quotation is typically derived from the sum of these costs plus a profit margin.
Laser processing services are predominantly billed based on machine time. While some providers opt for per-workpiece pricing, these rates are fundamentally calculated using the hourly operational costs of laser cutting.
The cost structure for laser cutting encompasses several key components:
- Power consumption
- Consumable parts wear and replacement
- Assist gas usage
- Machine depreciation
- Labor costs
It’s important to note that the total cost can vary significantly depending on factors such as laser power output, choice of assist gas, material type and thickness, and cutting complexity. For instance, high-power fiber lasers may have higher initial costs but offer faster cutting speeds and lower operational costs for certain materials compared to CO2 lasers.
To estimate specific laser cutting costs for your operations, you can refer to the comprehensive cost table provided below. For more precise calculations tailored to your unique setup and requirements, utilize our interactive laser cutting cost calculator.
By understanding and accurately calculating these costs, you can ensure competitive pricing while maintaining profitability in your laser cutting services.
1000W Laser Cutting Cost Table
Laser Power | Assisted Gas Consumption | Option I: Using Air Compressor Cutting Stainless Steel | Option II: Using O2 Cutting Stainless Steel | Option III:Using N2 Cutting Stainless Steel | |
---|---|---|---|---|---|
1000w | Power Consumption | Laser Power | 3 kw | 3 kw | 3 kw |
Water Chiller Group | 3.5 kw | 3.5 kw | 3.5 kw | ||
Main Machine | 6 kw | 6 kw | 6 kw | ||
Dust Exhausting Equipment | 3 kw | 3 kw | 3 kw | ||
Consumable Part | 0.38 USD /h | 0.38 USD /h | 0.38 USD /h | ||
Gas Consumption | 5.5 kw | approx 1.03 USD /h | approx 9.85 USD /h | ||
Total Power | 21 kw | 15.5 kw | 15.5 kw | ||
Average Power Consumption(Take 60% Cutting Efficiency) | 21×60%=12.6 kw | 15.5×60%=9.3 kw | 15.5×60%=9.3 kw | ||
All cost (0.15 USD/Kwh) | 2.23 USD/ h | 2.85 USD/ h | 11.66 USD/ h |
1500W Laser Cutting Cost Table
Laser Power | Assisted Gas Consumption | Option I: Using Air Compressor Cutting Stainless Steel | Option II: Using O2 Cutting Stainless Steel | Option III: Using N2 Cutting Stainless Steel | |
---|---|---|---|---|---|
1500w | Power Consumption | Laser Power | 4.5 kw | 4.5 kw | 4.5 kw |
Water Chiller Group | 3.5 kw | 3.5 kw | 3.5 kw | ||
Main Machine | 6 kw | 6 kw | 6 kw | ||
Dust Exhausting Equipment | 3 kw | 3 kw | 3 kw | ||
Consumable Part | 0.38 USD /h | 0.38 USD /h | 0.38 USD /h | ||
Gas Consumption | 5.5 kw | approx 1.03 USD /h | approx 9.85 USD /h | ||
Total Power | 22.5 kw | 17 kw | 17 kw | ||
Average Power Consumption(Take 60% Cutting Efficiency) | 22.5×60%=13.5 kw | 17×60%=10.2 kw | 17×60%=10.2 kw | ||
All cost (0.15 USD/Kwh) | 2.46 USD/ h | 2.85 USD/ h | 11.8 USD/ h |
2000W Laser Cutting Cost Table
Laser Power | Assisted Gas Consumption | Option I: Using Air Compressor Cutting Stainless Steel | Option II: Using O2 Cutting Stainless Steel | Option III: Using N2 Cutting Stainless Steel | |
---|---|---|---|---|---|
2000w | Power Consumption | Laser Power | 6 kw | 6 kw | 6 kw |
Water Chiller Group | 5.7 kw | 5.7 kw | 5.7 kw | ||
Main Machine | 6 kw | 6 kw | 6 kw | ||
Dust Exhausting Equipment | 3 kw | 3 kw | 3 kw | ||
Consumable Part | 0.38 USD /h | 0.38 USD /h | 0.38 USD /h | ||
Gas Consumption | 5.5 kw | approx 1.03 USD /h | approx 9.85 USD /h | ||
Total Power | 26.2 kw | 20.7 kw | 20.7 kw | ||
Average Power Consumption(Take 60% Cutting Efficiency) | 26.2×60%=15.72 kw | 20.7×60%=12.42 kw | 20.7×60%=12.42 kw | ||
All cost (0.15 USD/Kwh) | 2.8 USD/ h | 3.32 USD/ h | 12.14 USD/ h |
3000W Laser Cutting Cost Table
Laser Power | Assisted Gas Consumption | Option I: Using Air Compressor Cutting Stainless Steel | Option II: Using O2 Cutting Stainless Steel | Option III: Using N2 Cutting Stainless Steel | |
---|---|---|---|---|---|
3000w | Power Consumption | Laser Power | 9 kw | 9 kw | 9 kw |
Water Chiller Group | 5.7 kw | 5.7 kw | 5.7 kw | ||
Main Machine | 10 kw | 10 kw | 10 kw | ||
Dust Exhausting Equipment | 3 kw | 3 kw | 3 kw | ||
Consumable Part | 0.38 USD /h | 0.38 USD /h | 0.38 USD /h | ||
Gas Consumption | 5.5 kw | approx 1.03 USD /h | approx 9.85 USD /h | ||
Total Power | 33.2 kw | 27.7 kw | 27.7 kw | ||
Average Power Consumption(Take 60% Cutting Efficiency) | 33.2×60%=19.92 kw | 27.7×60%=16.62 kw | 27.7×60%=16.62 kw | ||
All cost (0.15 USD/Kwh) | 3.23 USD/ h | 3.78 USD/ h | 12.6 USD/ h |
4000W Laser Cutting Cost Table
Laser Power | Assisted Gas Consumption | Option I: Using Air Compressor Cutting Stainless Steel | Option II: Using O2 Cutting Stainless Steel | Option III: Using N2 Cutting Stainless Steel | |
---|---|---|---|---|---|
4000w | Power Consumption | Laser Power | 12 kw | 12 kw | 12 kw |
Water Chiller Group | 6 kw | 6 kw | 6 kw | ||
Main Machine | 10 kw | 10 kw | 10 kw | ||
Dust Exhausting Equipment | 3kw | 3 kw | 3 kw | ||
Consumable Part | 0.38 USD /h | 0.38 USD /h | 0.38 USD /h | ||
Gas Consumption | 5.5 kw | approx 0.97 USD /h | approx 9.14 USD /h | ||
Total Power | 36.5 kw | 31 kw | 31 kw | ||
Average Power Consumption(Take 60% Cutting Efficiency) | 36.5×60%=21.9 kw | 31×60%=18.6 kw | 31×60%=18.6 kw | ||
All cost (0.15 USD/Kwh) | 3.49 USD/ h | 3.97 USD/ h | 12.2 USD/ h |
6000W Laser Cutting Cost Table
Laser Power | Assisted Gas Consumption | Option I: Using Air Compressor Cutting Stainless Steel | Option II: Using O2 Cutting Stainless Steel | Option III: Using N2 Cutting Stainless Steel | |
---|---|---|---|---|---|
6000w | Power Consumption | Laser Power | 18 kw | 18 kw | 18 kw |
Water Chiller Group | 7.9kw | 7.9 kw | 7.9 kw | ||
Main Machine | 10 kw | 10 kw | 10 kw | ||
Dust Exhausting Equipment | 3 kw | 3 kw | 3 kw | ||
Consumable Part | 0.38 USD /h | 0.38 USD /h | 0.38 USD /h | ||
Gas Consumption | 5.5 kw | approx 1.03 USD /h | approx 9.85 USD /h | ||
Total Power | 44.4 kw | 38.9 kw | 38.9 kw | ||
Average Power Consumption(Take 60% Cutting Efficiency) | 44.4×60%=26.64 kw | 38.9×60%=23.34 kw | 38.9×60%=23.34 kw | ||
All cost (0.15 USD/Kwh) | 4.48 USD/ h | 5.01 USD/ h | 13.82 USD/ h |
8000W Laser Cutting Cost Table
Laser Power | Assisted Gas Consumption | Option I: Using Air Compressor Cutting Stainless Steel | Option II: Using O2 Cutting Stainless Steel | Option III: Using N2 Cutting Stainless Steel | |
---|---|---|---|---|---|
8000w | Power Consumption | Laser Power | 24 kw | 24 kw | 24 kw |
Water Chiller Group | 10kw | 10kw | 10kw | ||
Main Machine | 10 kw | 10 kw | 10 kw | ||
Dust Exhausting Equipment | 3 kw | 3 kw | 3 kw | ||
Consumable Part | 0.38 USD /h | 0.38 USD /h | 0.38 USD /h | ||
Gas Consumption | 5.5 kw | approx 1.03 USD /h | approx 9.14 USD /h | ||
Total Power | 52.5 kw | 47 kw | 47 kw | ||
Average Power Consumption(Take 60% Cutting Efficiency) | 52.5×60%=31.5 kw | 47×60%=28.2 kw | 47×60%=28.2 kw | ||
All cost (0.15 USD/Kwh) | 4.86 USD/ h | 5.34 USD/ h | 13.52 USD/ h |
10000W Laser Cutting Cost Table
Laser Power | Assisted Gas Consumption | Option I: Using Air Compressor Cutting Stainless Steel | Option II: Using O2 Cutting Stainless Steel | Option III: Using N2 Cutting Stainless Steel | |
---|---|---|---|---|---|
10000w | Power Consumption | Laser Power | 30kw | 30kw | 30kw |
Water Chiller Group | 10kw | 10kw | 10kw | ||
Main Machine | 10 kw | 10 kw | 10 kw | ||
Dust Exhausting Equipment | 3 kw | 3 kw | 3 kw | ||
Consumable Part | 0.38 USD /h | 0.38 USD /h | 0.38 USD /h | ||
Gas Consumption | 5.5 kw | approx 0.96 USD /h | approx 9.14 USD /h | ||
Total Power | 58.5 kw | 53 kw | 53 kw | ||
Average Power Consumption(Take 60% Cutting Efficiency) | 58.5×60%=35.1 kw | 53×60%=31.8 kw | 53×60%=31.8 kw | ||
All cost (0.15 USD/Kwh) | 4.86 USD/ h | 5.86 USD/ h | 14.0 USD/ h |
12000W Laser Cutting Cost Table
Laser Power | Assisted Gas Consumption | Option I: Using Air Compressor Cutting Stainless Steel | Option II: Using O2 Cutting Stainless Steel | Option III: Using N2 Cutting Stainless Steel | |
---|---|---|---|---|---|
12000w | Power Consumption | Laser Power | 36kw | 36kw | 36kw |
Water Chiller Group | 12kw | 12kw | 12kw | ||
Main Machine | 10 kw | 10 kw | 10 kw | ||
Dust Exhausting Equipment | 3 kw | 3 kw | 3 kw | ||
Consumable Part | 0.36 USD /h | 0.36 USD /h | 0.36 USD /h | ||
Gas Consumption | 5.5 kw | approx 0.96 USD /h | approx 9.14 USD /h | ||
Total Power | 66.5 kw | 61 kw | 61 kw | ||
Average Power Consumption(Take 60% Cutting Efficiency) | 66.5×60%=40 kw | 61×60%=36.6 kw | 61×60%=36.6 kw | ||
All cost (0.15 USD/Kwh) | 6.07 USD/ h | 6.54 USD/ h | 14.72 USD/ h |
15000W Laser Cutting Cost Table
Laser Power | Assisted Gas Consumption | Option I: Using Air Compressor Cutting Stainless Steel | Option II: Using O2 Cutting Stainless Steel | Option III: Using N2 Cutting Stainless Steel | |
---|---|---|---|---|---|
15000w | Power Consumption | Laser Power | 45kw | 45kw | 45kw |
Water Chiller Group | 15kw | 15kw | 15kw | ||
Main Machine | 10 kw | 10 kw | 10 kw | ||
Dust Exhausting Equipment | 3 kw | 3 kw | 3 kw | ||
Consumable Part | 0.36 USD /h | 0.36 USD /h | 0.36 USD /h | ||
Gas Consumption | 5.5 kw | approx 0.96 USD /h | approx 9.14 USD /h | ||
Total Power | 78.5 kw | 73 kw | 73 kw | ||
Average Power Consumption(Take 60% Cutting Efficiency) | 78.5×60%=47.1 kw | 73×60%=43.8 kw | 73×60%=43.8 kw | ||
All cost (0.15 USD/Kwh) | 7.09 USD/ h | 7.57 USD/ h | 15.76 USD/ h |
Understanding Laser Cutting
Laser cutting is a highly precise thermal cutting method that utilizes a focused, high-energy laser beam to melt, burn, or vaporize materials along a predetermined path. This advanced technology produces exceptionally clean cuts with minimal material waste, resulting in high-quality finished components.
Laser Cutting Processes
The laser cutting process employs three primary types of lasers, each optimized for specific applications:
- CO2 Lasers: Ideal for cutting, engraving, and boring non-metallic materials such as plastics, wood, and textiles. CO2 lasers are widely used due to their versatility, cost-effectiveness, and ability to produce high-quality cuts on organic materials.
- Nd:YAG (Neodymium-doped Yttrium Aluminum Garnet) Lasers: Suitable for both metallic and non-metallic materials, particularly effective on thicker substrates. Nd:YAG lasers offer high peak power and are often used in pulsed mode for precision cutting and drilling of metals.
- Fiber Lasers: The most advanced and efficient option, particularly for cutting reflective metals. Fiber lasers excel in speed, cut quality, and energy efficiency. Their solid-state design eliminates moving parts, resulting in lower maintenance requirements and increased reliability.
The choice of laser type depends on the material properties, required cutting speed, and desired finish quality.
Materials and Thickness Capabilities
Laser cutting technology can process a wide range of materials with varying thicknesses:
- Metals: Steel (mild, stainless, high-strength), aluminum alloys, titanium, brass, copper (up to 25mm thickness for some metals)
- Plastics: Acrylic, polycarbonate, ABS, nylon (up to 50mm for some polymers)
- Wood and composites: MDF, plywood, carbon fiber reinforced plastics (up to 50mm)
- Glass and ceramics: Specialized laser systems required (up to 10mm)
- Fabrics and textiles: Natural and synthetic materials (multiple layers possible)
Material thickness capabilities depend on the laser power, wavelength, and material properties. Generally, increasing material thickness requires higher laser power or reduced cutting speeds to maintain cut quality.
Benefits of Laser Cutting
Laser cutting offers numerous advantages over conventional mechanical cutting methods:
- Unparalleled Precision: Laser beams maintain consistent focus and energy, enabling cuts with tolerances as tight as ±0.1mm.
- Design Flexibility: Easily produces complex geometries, intricate patterns, and fine details that would be challenging or impossible with traditional methods.
- High-Speed Processing: Significantly faster than many conventional cutting techniques, especially for intricate designs.
- Superior Edge Quality: Produces clean, smooth cuts with minimal burring, often eliminating the need for secondary finishing operations.
- Minimal Heat Affected Zone (HAZ): Localized heat input reduces thermal distortion and material property changes in the workpiece.
- Non-Contact Process: Eliminates tool wear and associated maintenance costs.
- Material Efficiency: Nested cutting patterns minimize material waste, optimizing resource utilization.
- Automation Potential: Easily integrated into automated production lines for increased efficiency and consistency.
Factors Influencing Laser Cutting Cost
The cost of laser cutting is a multifaceted consideration, influenced by various factors ranging from material selection to design intricacy. A comprehensive understanding of these elements enables more accurate budgeting and potential cost optimizations.
Material Costs
Material Type: The cost spectrum varies significantly across materials, with metals generally commanding higher prices than plastics. For instance, stainless steel and titanium are typically more expensive to cut than mild steel or aluminum.
Material Thickness: Thicker materials often incur higher costs due to increased laser power requirements and reduced cutting speeds. The relationship between thickness and cost is not linear; beyond certain thresholds, costs can increase exponentially.
Machine Operating Costs
Laser Power and Type: Higher-power lasers, while more expensive to operate due to increased energy consumption, can process materials faster. Fiber lasers generally have lower operating costs compared to CO2 lasers for metal cutting applications.
Maintenance and Consumables: Regular maintenance, including optics cleaning, lens replacement, and assist gas management, is crucial for optimal performance. These ongoing costs can significantly impact overall operational expenses.
Labor Costs
Setup and Programming Time: The time required for machine setup, CNC programming, and nesting optimization contributes to labor costs. Advanced CAM software can reduce setup times but may require higher-skilled operators.
Operator Expertise: Skilled operators can enhance efficiency through optimized cutting parameters and troubleshooting abilities, potentially justifying their higher wages through improved productivity and reduced material waste.
Design Complexity
Geometric Intricacy: Complex designs with numerous tight corners, small features, or intricate patterns require more time and precision, increasing processing time and cost. Advanced nesting algorithms can optimize material usage for complex parts.
File Preparation and Engineering: Significant pre-processing, including design optimization for laser cutting, DXF file cleanup, and lead-in/lead-out placement, can impact overall costs but may result in improved cut quality and efficiency.
Production Volume
Economies of Scale: Larger order quantities typically reduce per-unit costs due to amortized setup times and optimized material utilization. However, the relationship is not always linear and may plateau at certain volumes.
Recurring Orders: Regular, repeat orders can lead to cost reductions through streamlined processes, optimized toolpaths, and potentially negotiated material prices. Implementing standardized procedures for recurring jobs can further enhance efficiency.
Additional Considerations:
- Assist Gas Selection: The choice between nitrogen, oxygen, or compressed air as assist gas can significantly impact both cost and cut quality.
- Post-Processing Requirements: Additional operations like deburring or heat treatment can add to the overall cost but may be necessary for certain applications.
- Machine Utilization: Higher machine utilization rates can lead to lower overhead costs per part, emphasizing the importance of efficient job scheduling.
Calculating Laser Cutting Costs
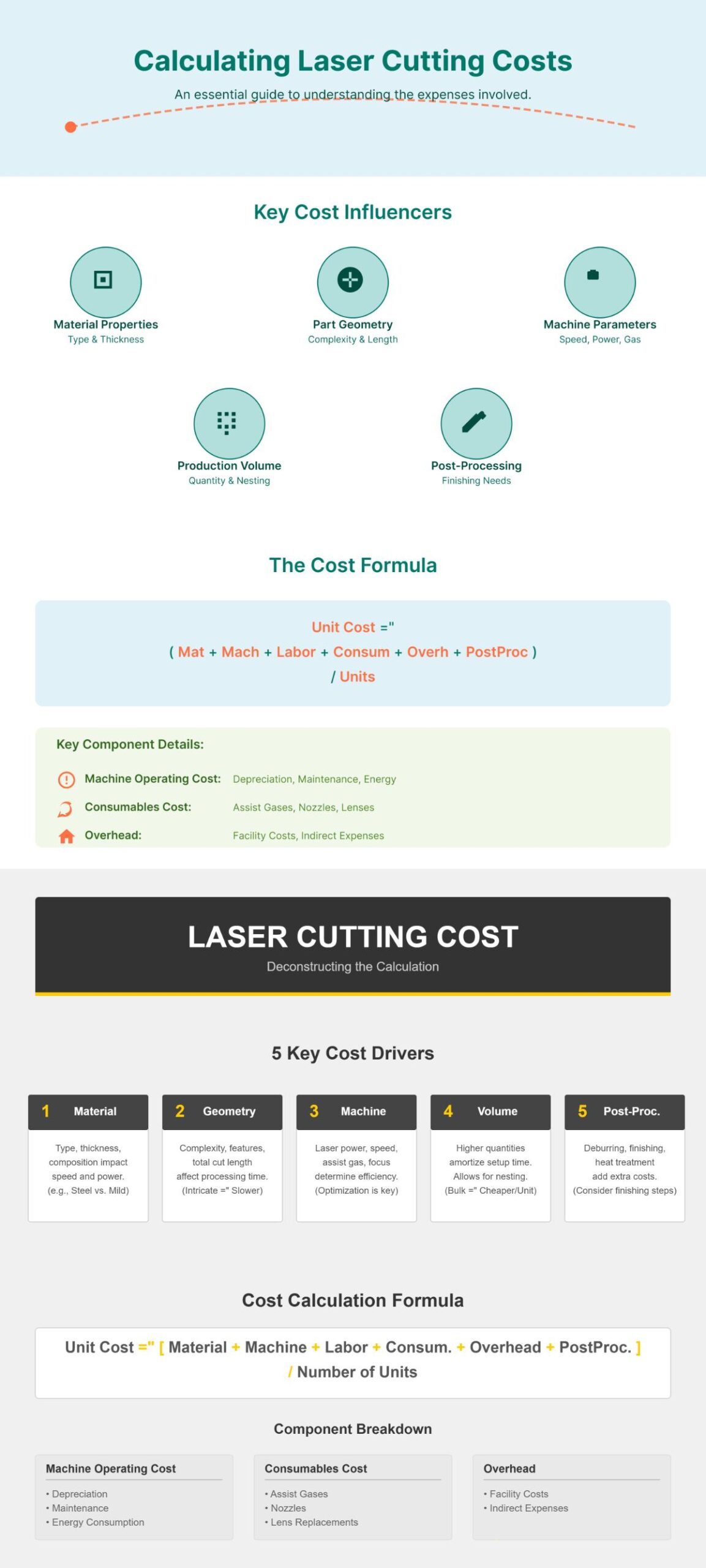
When assessing the cost of laser cutting, it’s essential to understand both the per-unit expenses and software tools available for accurate estimation.
Per-Unit Cost Calculation
To calculate the per-unit cost of laser cutting, one must consider multiple factors:
Material Properties: Type, thickness, and composition affect cutting speed and consumable usage. For example, stainless steel requires more laser power and assist gas than mild steel of the same thickness.
Part Geometry: Complexity, feature size, and total cut length influence processing time. Intricate designs with tight tolerances may require slower cutting speeds and multiple passes.
Machine Parameters: Laser power, cutting speed, assist gas type/pressure, and focal length optimization impact both quality and efficiency.
Production Volume: Higher quantities often reduce per-unit costs due to setup time amortization and potential for nesting optimization.
Post-Processing Requirements: Deburring, surface finishing, or heat treatment needs can add significant costs.
A comprehensive per-unit cost calculation formula would be:
Per-Unit Cost = (Material Cost + Machine Operating Cost + Labor Cost + Consumables Cost + Overhead + Post-Processing Cost) / Number of Units
Where:
- Machine Operating Cost includes depreciation, maintenance, and energy consumption
- Consumables Cost covers assist gases, nozzles, and lens replacements
- Overhead accounts for facility costs and indirect expenses
Software for Cost Estimation
Advanced laser cutting cost estimation software enhances precision and efficiency through:
Real-time Data Integration: Incorporates live material prices, machine utilization rates, and energy costs.
Comprehensive Process Modeling: Simulates the entire cutting process, considering beam characteristics, heat affected zone, and kerf width for accurate time and quality predictions.
Nesting Optimization: Maximizes material utilization through intelligent part placement algorithms.
Machine Learning Capabilities: Continuously improves estimations based on historical job data and actual production outcomes.
Multi-variable Optimization: Balances cost, quality, and lead time to suggest optimal process parameters.
CAD/CAM Integration: Directly imports part geometries for accurate feature recognition and costing.
Customizable Reporting: Generates detailed cost breakdowns and quotes tailored to specific customer requirements.
Selecting and implementing the right software solution is crucial for maintaining competitiveness and profitability in the laser cutting industry, especially as materials and technologies continue to evolve.
Reducing Laser Cutting Expenses
Cost efficiency in laser cutting is achievable through a synergistic approach combining intelligent design strategies, optimal material selection, and streamlined production processes. These targeted methodologies are crucial for minimizing expenses while maintaining high-quality output.
Design Optimization
Effective design optimization plays a pivotal role in cost reduction. Streamlined layouts with optimized cut paths can significantly reduce machine time and associated costs. Designers can decrease piece costs by meticulously analyzing design elements such as cut geometry, nesting efficiency, and part orientation. Implementing advanced CAD/CAM software solutions that offer automatic nesting and toolpath optimization can improve design efficiency while reducing reliance on costly proprietary programs. Additionally, considering design for manufacturability (DFM) principles early in the process can prevent costly revisions and production issues.
Material Selection
The choice of materials can dramatically influence overall costs. Utilizing recycled or lower-grade materials where appropriate can offer substantial cost advantages without compromising structural integrity. It’s essential to conduct a comprehensive analysis of material properties, including thermal conductivity, reflectivity, and thickness tolerance, to ensure optimal laser cutting performance. Balancing material cost with machinability and end-use requirements is crucial. Establishing strong relationships with suppliers and leveraging bulk purchasing power can lead to preferential pricing on high-quality materials.
Efficient Production Practices
Implementing lean manufacturing principles and efficient production practices is vital for cost reduction in laser cutting operations. Optimizing machine parameters such as cutting speed, power output, and assist gas pressure for each material type can significantly improve throughput and reduce operational costs. Leveraging economies of scale through strategic production scheduling and batch processing of similar materials or thicknesses minimizes setup times and maximizes machine utilization.
Regular preventive maintenance, including proper alignment of optics and cleanliness of the cutting head, ensures consistent cut quality and reduces costly downtime. Implementing real-time monitoring systems can help identify and address inefficiencies promptly. Additionally, investing in operator training and developing standard operating procedures (SOPs) can lead to improved productivity and reduced waste.
Exploring alternative cutting technologies, such as fiber lasers for thin materials or waterjet cutting for thick or highly reflective materials, may offer cost advantages for specific applications. Finally, establishing long-term partnerships with laser cutting service providers can result in volume discounts and priority scheduling, further reducing overall production costs.
Comparative Cost Analysis
In assessing the financial implications of laser cutting, it’s crucial to compare it with traditional and alternative cutting methods in terms of efficiency and cost-effectiveness.
Laser Cutting vs. Traditional Cutting Methods
Laser cutting technology has revolutionized metal fabrication, offering distinct advantages over traditional mechanical cutting methods such as sawing, shearing, and punching. This advanced process utilizes a high-powered laser beam to melt, vaporize, or blow away material with exceptional precision and speed, often surpassing conventional techniques in efficiency and quality.
In terms of precision, laser cutting achieves tolerances as tight as ±0.1 mm, significantly outperforming most traditional methods. This high accuracy reduces the need for secondary finishing operations, streamlining production workflows. Moreover, laser cutting’s non-contact nature minimizes material deformation and eliminates tool wear, ensuring consistent quality over long production runs.
Regarding speed, modern fiber laser systems can cut thin sheet metal at rates up to 60 meters per minute, dramatically outpacing traditional methods. This rapid processing, combined with minimal setup times and the ability to quickly switch between different cutting patterns, results in substantially reduced labor costs and increased throughput.
Material utilization is another key advantage of laser cutting. Its narrow kerf width (as small as 0.1 mm for thin materials) and ability to nest parts closely together can reduce material waste by up to 30% compared to traditional methods. This efficiency is particularly valuable when working with expensive alloys or large production volumes.
However, the initial investment for a laser cutting system can be significant, often ranging from $300,000 to over $1 million for high-power machines. Additionally, operating costs, including energy consumption (typically 10-30 kW for industrial systems) and specialized maintenance, are higher than those for traditional equipment.
Traditional cutting methods, while generally slower and less precise, still maintain relevance in certain applications. They often have lower initial costs, simpler operation, and can be more suitable for very thick materials or in environments where laser safety concerns or power availability are issues.
Laser Cutting vs. Waterjet Cutting
Waterjet cutting employs a high-pressure stream of water, typically mixed with abrasive particles, to erode and cut through materials. The comparison between laser and waterjet cutting centers on cut quality, operational costs, and material compatibility. Laser cutting excels in precision, delivering superior edge quality with a minimal heat-affected zone (HAZ), making it ideal for intricate designs and thin materials. Conversely, waterjet cutting’s cold-cutting process eliminates heat generation, significantly reducing the risk of thermal distortion, work hardening, or changes in material properties.
Waterjet cutting demonstrates greater versatility, effectively processing a wider range of materials, including metals, composites, stone, and ceramics. It can handle thicker sections (up to 200 mm in some materials) and harder substances that may prove challenging or cost-prohibitive for laser cutting. The absence of heat also allows waterjet to cut heat-sensitive materials without compromising their integrity.
Operational costs vary based on application. Laser cutting generally offers higher cutting speeds and lower operating costs for thin materials, particularly metals. However, as material thickness increases, waterjet cutting becomes more economical due to its consistent cutting speed across varying thicknesses. Laser cutting thick materials often requires multiple passes or increased power, leading to higher energy consumption and potentially reduced consumable life.
The choice between these technologies depends on specific project requirements, including material type, thickness, desired edge quality, production volume, and overall cost considerations. Many fabrication shops utilize both technologies to optimize their manufacturing capabilities and flexibility.
Feature | Laser Cutting | Waterjet Cutting |
---|---|---|
Precision | High | Medium |
Cut Quality | Excellent | Good |
Material Distortion | Minimal | None |
Equipment Cost | Higher | High |
Operational Speed | Fast | Moderate |
Energy Consumption | High | Medium |
Maintenance Cost | Variable | High |
Laser Cutting vs. Plasma Cutting
Laser cutting and plasma cutting are two prominent thermal cutting processes in metal fabrication, each with distinct advantages for specific applications. Laser cutting excels in precision and produces cleaner cuts with a minimal heat-affected zone (HAZ), making it ideal for intricate designs and thin to medium-thickness materials. This process utilizes a focused beam of light to melt or vaporize material, resulting in exceptionally smooth edge quality and tight tolerances, often within ±0.1mm.
In contrast, plasma cutting leverages a high-temperature ionized gas to melt through conductive materials, making it particularly effective for cutting thick metal sheets, typically up to 50mm for high-definition systems. While plasma cutting generally offers faster cutting speeds on thicker materials and lower initial equipment and operational costs, it yields lower precision compared to laser cutting, with tolerances typically around ±0.5mm.
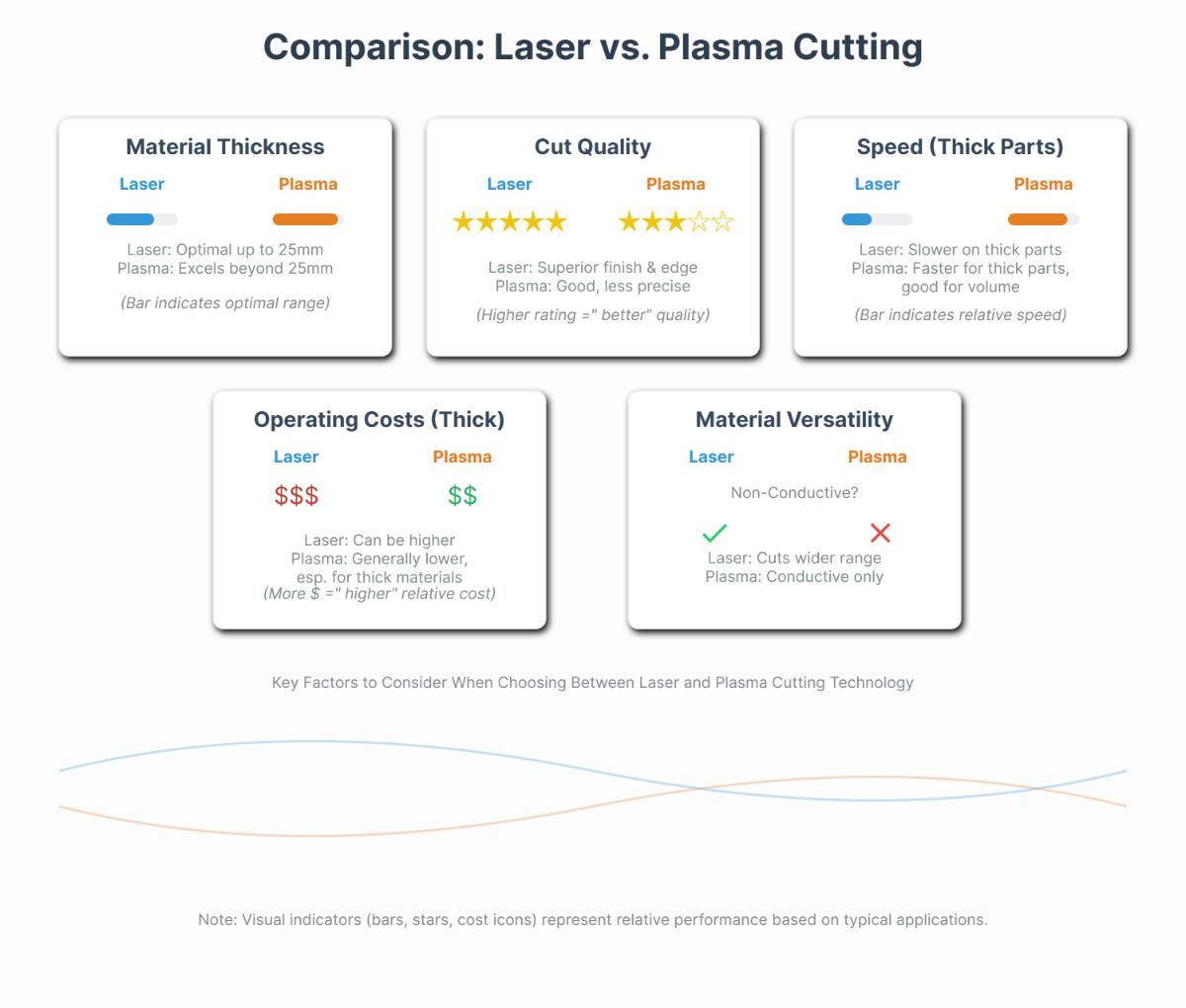
The choice between these technologies often depends on specific project requirements:
- Material thickness: Laser cutting is optimal for materials up to 25mm thick, while plasma excels beyond this range.
- Cut quality: Laser cutting provides superior surface finish and edge quality, crucial for components requiring minimal post-processing.
- Production volume: Plasma cutting’s higher speed can be advantageous for large-scale production of thick parts.
- Operating costs: Plasma systems generally have lower running costs, especially for thicker materials.
- Material versatility: Lasers can cut a wider range of materials, including non-conductive ones, whereas plasma is limited to conductive materials.
Advancements in both technologies, such as fiber lasers and high-definition plasma systems, continue to narrow the performance gap, offering fabricators more flexible and efficient cutting solutions across various applications.
Feature | Laser Cutting | Plasma Cutting |
---|---|---|
Precision | High | Moderate |
Material Thickness | Medium | High |
Cut Speed (Thick Material) | Moderate | Fast |
Surface Finish | Smooth | Rougher |
Equipment Cost | Higher | Lower |
Operational Cost | Medium | Lower |